NCBI Bookshelf. A service of the National Library of Medicine, National Institutes of Health.
Jamison DT, Breman JG, Measham AR, et al., editors. Disease Control Priorities in Developing Countries. 2nd edition. Washington (DC): The International Bank for Reconstruction and Development / The World Bank; 2006. Co-published by Oxford University Press, New York.
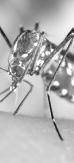
Malaria is the most important of the parasitic diseases of humans, with 107 countries and territories having areas at risk of transmission containing close to 50 percent of the world's population (Hay and others 2004; WHO 2005). More than 3 billion people live in malarious areas and the disease causes between 1 million and 3 million deaths each year (Breman, Alilio, and Mills 2004; Snow and others 2003). Recent estimates of the global falciparum malaria morbidity burden have increased the number to 515 million cases, with Africa suffering the vast majority of this toll (Snow and others 2005). In addition, almost 5 billion clinical episodes resembling malaria occur in endemic areas annually, with more than 90 percent of this burden occurring in Africa (Breman 2001; Breman, Alilio, and Mills 2004; Carter and Mendis 2002; Snow and others 1999, 2003; Snow, Trape, and Marsh 2001).
The disease has resurged in many parts of the tropics, and nonmalarious countries face continual danger from importation. Contributing to this resurgence are the increasing problems of Plasmodium falciparum resistance to drugs and of the Anopheles vectors' resistance to insecticides. The recent findings that insecticide-treated nets (ITNs) are extremely cost-effective in preventing malaria and overall deaths and that intermittent preventive therapy (IPT) (treatment doses given during periods of vulnerability) is effective for protecting pregnant women and their fetuses, along with the discovery of new drugs (artemisinins) and their use in combination with other antimalarials and promising vaccine trials, have given great impetus to the battle against this scourge (Alonso and others 2004; Armstrong-Schellenberg and others 2001; Lengeler 2004; Newman and others 2003; Yeung and others 2004).
Causes, Epidemiology, Manifestations, and Diagnosis
Four species of the genus Plasmodium cause malarial infections in humans: P. falciparum, P. vivax, P. ovale, and P. malariae. Virtually all deaths are caused by falciparum malaria. Human infection begins when the malaria vector, a female anopheline mosquito, inoculates plasmodial sporozoites from its salivary gland into humans during a blood meal. The sporozoites mature in the liver and are released into the bloodstream as merozoites. These invade red blood cells, causing malaria fevers. Some forms of the parasites (gametocytes) are ingested by anopheline mosquitoes during feeding and develop into sporozoites, restarting the cycle.
P. falciparum predominates in Haiti, Papua New Guinea, and Sub-Saharan Africa, while P. vivax is more common in Central America and the Indian subcontinent and causes more than 80 million clinical episodes of illness yearly (Mendis and others 2001). The prevalence of these two species is approximately equal in the Indian subcontinent, eastern Asia, Oceania, and South America. P. malariae is found in most endemic areas, especially throughout Sub-Saharan Africa, but is much less common than the other species. P. ovale is unusual outside Africa, and where it is found accounts for less than 1 percent of isolates.
While more than 40 anophelines can transmit malaria, the most effective are those such as Anopheles gambiae, which are long-lived, occur in high densities in tropical climates, breed readily, and bite humans in preference to other animals. The entomological inoculation rate (EIR)—that is, the number of sporozoite-positive mosquito bites per person per year—is the most useful measure of malarial transmission and varies from less than 1 in some parts of Latin America and Southeast Asia to more than 300 in parts of tropical Africa.
The epidemiology of malaria may vary considerably within relatively small geographic areas. In tropical Africa or coastal Papua New Guinea, with P. falciparum transmission, more than one human bite per infected mosquito can occur per day and people are infected repeatedly throughout their lives. In such areas, morbidity and mortality during early childhood are considerable. For survivors, some immunity against disease develops in these areas, and by adulthood, most malarial infections are asymptomatic. This situation, with frequent, intense, year-round transmission, is termed stable malaria. In areas where transmission is low, erratic, or focal, full protective immunity is not acquired and symptomatic disease may occur at all ages. This situation is termed unstable malaria. An epidemic or complex emergency can develop when changes in environmental, economic, or social conditions occur, such as heavy rains following drought or migrations of refugees or workers from a nonmalarious to an endemic region. A breakdown in malaria control and prevention services intensifies epidemic conditions. Epidemics occur most often in areas with unstable malaria, such as Ethiopia, northern India, Madagascar, Sri Lanka, and southern Africa. Many other African countries situated in the Sahelian and Sub-Saharan areas are susceptible to epidemics (Djimdé and others 2004; Worrall, Rietveld, and Delacollette 2004). Public health specialists have only recently begun to appreciate the considerable contribution of urban malaria, with up to 28 percent of the burden in Africa occurring in rapidly growing urban centers (Keiser and others 2004).
The determinants of malaria and of risk factors for patients and communities relate to intrinsic (human, parasite, and vector) and extrinsic (environmental, control, and socioeconomic) factors (figure 21.1)(Breman 2001). Both humoral and cellular immunity are necessary for protection.

Figure 21.1
Determinants of the Malaria Burden: Intrinsic and Extrinsic Factors
Anemia may be quite common among young children living in areas with stable transmission, particularly where the parasite is resistant to chloroquine, sulfadoxine-pyrimethamine (SP), or other drugs. Correctly and promptly treated, uncomplicated falciparum malaria has a mortality rate of approximately 0.1 percent (Sudre and others 1992). Once vital organ dysfunction occurs or the proportion of erythrocytes infected increases to more than 3 percent, mortality rises steeply. Coma is a characteristic and ominous feature of falciparum malaria, and despite treatment it is associated with death rates of some 20 percent among adults and 15 percent among children. Convulsions, usually generalized and often repeated, occur in up to 50 percent of children with cerebral malaria (CM) (Mung'Ala-Odera, Snow, and Newton 2004). Whereas less than 3 percent of adults suffer neurological sequelae, roughly 10 to 15 percent of children surviving CM—especially those with hypoglycemia, severe malarial anemia (SMA), repeated seizures, and deep coma—have some residual neurological deficit when they regain consciousness. Protein-calorie under-nutrition and micronutrient deficiencies, particularly zinc and vitamin A, contribute substantially to the malaria burden (Caulfield, Richard, and Black 2004).
In areas with intense and stable transmission, falciparum malaria in primigravid and secundigravid women is associated with low birthweight (average reduction of about 170 grams) and consequently with increased infant and childhood mortality (Steketee and others 2001). HIV infection predisposes all pregnant women to more frequent and severe malaria, and the reverse may also be true (Ter Kuile and others 2004). P. vivax malaria in pregnancy is also associated with a reduction in birthweight (average reduction of some 100 grams), and this effect is greater in multigravid than in primigravid women (Nosten and others 1999).
The confirmatory diagnosis of clinical malaria rests on the microscopic demonstration of asexual forms of the parasite in stained peripheral blood smears. Newer diagnostic tests using antigen and nucleic acid detection methods are being evaluated. These tests are promising, but their limitations in relation to species sensitivity (except for P. falciparum), parasite quantitation, field feasibility, and costs necessitate further development and evaluation (Warhurst and Williams 2004).
Burden of Disease
In 2001, the World Health Organization (WHO) ranked malaria as the eighth-highest contributor to the global disease burden as reflected in disability-adjusted life years (DALYs), and the second highest in Africa (WHO 2002a). The DALYs attributable to malaria were estimated largely from the effects of P. falciparum infection as a direct 0000cause of death and the much smaller contributions of short-duration, self-limiting, or treated mild febrile events, including malaria-specific mild anemia and neurological disability following CM (Murray and Lopez 1996, 1997). The estimate assumes that each illness event or death can be attributed only to a single cause that can be measured reliably. Table 21.1 shows deaths and DALYs from deaths attributable to malaria and to all causes by WHO region (WHO 2002a). It does not include the considerable toll caused by the burden of malaria-related moderate and severe anemia, low birthweight, and comorbid events (Snow and others 2003). Sub-Saharan African children under four represent 82 percent of all malaria-related deaths and DALYs. Malaria accounts for 2.0 percent of global deaths and 2.9 percent of global DALYs. In the African region of WHO, 9.0 percent of deaths and 10.1 percent of DALYs are attributable to malaria.
Table 21.1
Deaths and DALYs from Deaths Attributable to All Causes and to Malaria by WHO Region, 2000.
Recent analysis of falciparum malaria morbidity concludes that 515 (interquartile range 298 to 659) million cases occur yearly (table 21.2). This figure is 92 percent higher than the 278 million malaria cases estimated by WHO for 1998, which also includes those attributable to P. vivax, and 200 percent higher than previous estimates for areas outside of Africa (Snow and others 2005). While the malaria incidence globally is 236 episodes per 1,000 persons per year in all endemic areas, it ranges from about 400 to 2,000 (median 830) episodes per 1,000 persons per year in areas with intense, stable (hyperendemic and holoendemic) transmission; these areas represent 38 percent of all falciparumendemic areas.
Table 21.2
Population at Risk for Falciparum Malaria, Cases and Attack Rates by World Health Organization Region, 2002*.
Background
Recent estimates of malaria deaths have varied from 0.5 million to 3.0 million per year (Breman 2001; Breman, Alilio, and Mills 2004; Snow and others 2003). Of the 10.6 million yearly deaths in children younger than 5 years 8 percent are ascribed to malaria (Bryce and others 2005). In 1998, an empirical analysis of malaria mortality undertaken on behalf of WHO used malaria risk maps to capture measures of disability, morbidity, and mortality associated with P. falciparum prevalence rates among African populations and yielded an estimate of about 1 million (Korenromp and others 2003; Snow and Marsh 2002; Snow and others 2003; Snow, Trape, and Marsh 2001). Each malarious country must now measure its own burden and progress toward decreasing that burden (WHO 2005).
Geographic and climate-driven (mainly rainfall) models of suitability for malaria transmission characterize the diversity of malaria transmission across the African continent (Craig, Snow, and le Sueur 1999; Snow and others 1999). Four distinct areas can be identified:
- class 1, no transmission (northern and parts of southern Africa)
- class 2, marginal risk (mainly in some areas of southern Africa and in high-altitude [>1500 meters] settings)
- class 3, seasonal transmission with epidemic potential (along the Sahara fringe and in highlands)
- class 4, stable and unstable malarious areas (most areas south of the Sahara to southern Africa and below an altitude of around 1,500 meters).
Direct Consequences of P. falciparum Infection
Two major syndromes, CM and moderate (hemoglobin less than 11 grams per deciliter) and severe (hemoglobin less than 8 grams per deciliter) malarial anemia, contribute directly and significantly to malaria mortality (tables 21.3 and 21.4). The differences in numbers derive from the different methods of calculating the burden. Children presenting with an acute febrile disease, peripheral parasitemia, and low hemoglobin concentrations account for the majority of inpatient admissions in areas with stable transmission. The arbitrary definition of 5 grams of hemoglobin per deciliter is prognostic for a fatal outcome and proves useful clinically as a criterion for transfusion. Lactic acidosis commonly coexists with hypoglycemia and is (with coma, repeated convulsions, shock, and hyperparasitemia) an important predictor of death from severe malaria (White and Breman 2005; WHO 2000b).
Table 21.3
Malaria-related Mortality and Morbidity, Africa, 2000.
Table 21.4
Deaths from Malaria in Children Under Five, Africa, 2001.
The vast majority of deaths in developing countries occur outside the formal health service, and in Africa, most government civil registration systems are incomplete (Breman 2001; Greenberg and others 1989). Newer demographic and disease-tracking systems are being used globally and should help rectify the woefully inadequate vital statistics available for malaria and other diseases (INDEPTH 2002).
Health personnel usually attribute causes of death during demographic surveillance system surveys through a verbal autopsy interview with relatives of the deceased about the symptoms and signs associated with the terminal illness. Both the specificity and the sensitivity of verbal autopsy vary considerably depending on the background spectrum of other common diseases, such as acute respiratory infection, gastroenteritis, and meningitis, which share common clinical features with malaria (Korenromp and others 2003).
In malarious Africa, some 30 to 60 percent of outpatients with fever may have parasitemia. Monthly surveillance of households will detect a quarter of the medical events that are detected through weekly surveillance, and weekly contacts with cohorts identify approximately 75 percent of events detected through daily surveillance (Snow, Menon, and Greenwood 1989). Given the predominance of fevers, malaria case management in Africa and other endemic areas usually centers on presumptive diagnosis.
Estimates of the frequency of fever among children suggest one episode every 40 days. If we assume that the perceived frequency of fever in Africa is similar across all transmission areas (and possibly all ages), African countries would witness approximately 4.9 billion febrile events each year. Estimates indicate that in areas of stable malaria risk, a minimum of 2.7 billion exposures to antimalarial treatment will occur each year for parasitemic persons, or 4.93 per person per year (Snow and others 2003). While these diagnostic, patient management, and drug delivery assumptions are debatable, they indicate the magnitude of the challenges malaria presents.
The case-fatality rates of CM are high, even with optimal management. Murphy and Breman (2001) report a mean case-fatality rate of 19.2 percent and Snow and others (2003) cite a figure of 17.5 percent. Those who succumb at home without optimal treatment will have higher case-fatality rates.
Studies of neurological sequelae after severe malaria indicated that 3 to 28 percent of survivors suffered from such sequelae, including prolonged coma and seizures (MungAla-Odera, Snow, and Newton 2004). CM is associated with hemiparesis, quadriparesis, hearing and visual impairments, speech and language difficulties, behavioral problems, epilepsy, and other problems (table 21.3). The incidence of neurocognitive sequelae following severe malaria is only a fraction of the true residual burden, and the impact of milder illness is unknown.
Studies of children presenting to hospital with malaria and hemoglobin of less than or equal to 5 grams per deciliter indicate a median transfusion rate of 80.1 percent. Thus, 275,400 to 442,290 surviving SMA admissions, newborn through 14 years, will be exposed to blood transfusion each year in Sub-Saharan Africa. As a result, each year 5,300 to 8,500 children, age birth through 14 years, living in stable endemic areas of Africa are likely to acquire HIV infection because of exposure to blood transfusion to manage SMA (Colebunders and others 1991; Savarit and others 1992).
Indirect and Comorbid Risks
The DALY model of malaria does not sufficiently take it into account as an indirect cause of broader morbid risks. Some consider anemia to be caused indirectly unless linked to acute, high-density parasitemia. Similarly, low birthweight may also be indirectly attributable to malaria, and a child's later under-nutrition and growth retardation linked to malaria infection enhances the severity of other concomitant or comorbid infectious diseases through immune suppression. Thus, malaria infection contributes to broad causes of mortality beyond the direct fatal consequences of infection and is probably underestimated (Breman, Alilio, and Mills 2004; Snow and others 2003).
In Africa, pregnant women experience few malaria-specific fever episodes but have an increased risk of anemia and placental sequestration of the parasite. Maternal clinical manifestations are more apparent in areas with less intense transmission, particularly in Asia. Estimates indicate that in Sub-Saharan Africa, malaria-associated anemia is responsible for 3.7 percent of maternal mortality, or approximately 5,300 maternal deaths annually.
Prematurity and intrauterine growth retardation resulting in low birthweight associated with maternal malaria account for 3 to 8 percent of infant mortality in Africa (Steketee and others 1996, 2001). Assuming an infant mortality rate of 105 per 1,000 live births, Snow and others (2003) calculate that in 2000, 71,000 to 190,000 infant deaths were attributable to malaria in pregnancy (table 21.3). Other studies indicate that malaria-associated low birthweight accounted for 62,000 to 363,000 infant deaths (Murphy and Breman 2001).
Anemia among African children is caused by a combination of nutritional deficiencies and iron loss through helminth infection, red cell destruction, decreased red cell production as a result of infectious diseases, and genetically determined hemoglobinopathies. Chronic or repeated infections, often associated with parasite resistance to drugs, are more likely to involve bone marrow suppression (Menendez, Fleming, and Alonso 2000).
Murphy and Breman (2001) estimate that 190,000 to 974,000 deaths per year in Sub-Saharan Africa are attributable to SMA. Children residing in areas where the prevalence of P. falciparum was more than 25 percent had a 75 percent prevalence of anemia. By modeling the relationship between anemia and parasite prevalence, Snow and others (2004) found that mild anemia rose 6 percent for every 10 percent increase in the prevalence of infection. Reducing the incidence of new infections through ITNs or the prevalence of blood-stage infections through chemoprophylaxis or IPT for children halved the risk of anemia.
Caulfield, Richard, and Black (2004) report that iron, zinc, and protein-calorie deficits are responsible for a considerable amount of malaria-related mortality and morbidity and indicate that 57.3 percent of deaths of underweight children under five are attributable to nutritional deficiencies. One striking feature of the global distribution of anthropometric markers of undernutrition is its congruence with the distribution of endemic malaria. Improved growth among young children has more recently been demonstrated in The Gambia and Kenya in a comparison of those protected and unprotected by ITNs (Ter Kuile and others 2003).
Early during the HIV epidemic, Greenberg and others (1988) and Greenberg (1992) demonstrated that malaria-associated anemia treated with unscreened blood transfusions contributed to HIV transmission. At the same time, two longitudinal cohort studies in Kenya and Uganda and one hospital-based case-control study in Uganda demonstrated that HIV infection approximately doubles the risk of malaria parasitemia and clinical malaria in nonpregnant adults and that increased HIV immunosuppression is associated with higher-density parasitemias (French and others 2001; Whitworth and others 2000). In pregnant women, the presence of HIV increases the rate and intensity of parasitemia and frequency of anemia (Ter Kuile and others 2004). The increasing incidence of HIV-associated febrile illnesses may lead to increased use of antimalarials. Some believe that the recommended use of trimethoprim-sulfamethoxazole for prophylaxis of bacterial pneumonia and other infections in HIV/AIDS patients may contribute to SP resistance and that monitoring is required. Yet, evaluation of trimethoprim-sulfamethoxazole for malaria prophylaxis in Mali did not show any increases in parasite resistance mutations specific for these drugs (Thera and others 2005).
Malaria accounts for 13 to 15 percent of medical reasons for absenteeism from school, but little information is available on the performance of parasitized schoolchildren (Holding and Kitsao-Wekulo 2004). A randomized placebo control study of chloroquine prophylaxis in Sri Lankan schoolchildren demonstrated an improvement in mathematics and language scores by those who received chloroquine but found no difference in absenteeism (Fernando and others 2003) As noted earlier, malaria may result in low birthweight, and low birthweight can lead to a range of persistent impaired outcomes, predominantly behavioral difficulties, cerebral palsy, mental retardation, blindness, and deafness. The recently launched studies of intermittent preventive treatments during infancy (IPTi) should provide a more precise means of examining the benefits of IPTi and consequences on learning and performance of infection early in life (Holding and Kitsao-Wekulo 2004; Rosen and Breman 2004; Schellenberg and others 2005).
Interventions and Their Effectiveness
Malaria will be conquered only by full coverage, access to, and use of antimalarial services by priority groups; rapid, accurate diagnosis; prompt and effective patient management (diagnosis, treatment, counseling and education, referral); judicious use of insecticides to kill and repel the mosquito vector, including the use of ITNs; and control of epidemics. Eliminating malaria from most endemic areas remains a distant, huge, but surmountable challenge because of the widespread Anopheles breeding sites; the large number of infected people; the use of ineffective antimalarial drugs; and the inadequacies of resources, infrastructure, and control programs. The Roll Back Malaria Partnership, which began in 1998, aims to halve the burden of malaria by 2010 and has developed strategies and targets for 2005 (box 21.1). While ambitious, the initiative is making substantial progress by means of effective and efficient deployment of currently available interventions (WHO 2003, 2005). Indeed, Brazil, Eritrea, India, and Vietnam are reporting recent successes in reducing the malaria burden (Barat 2005). Despite the enormous investment in developing a malaria vaccine administered by means of a simple schedule and recent promising results in the laboratory and in field trials in Africa, no effective, long-lasting vaccine is likely to be available for general use in the near future (Alonso and others 2004; Ballou and others 2004).
Drug Use
Proper use of drugs is essential. Early diagnosis and effective treatment of patients lends credibility to the malaria program, strengthens confidence in the health care system by families and communities, and raises the esprit of clinicians and public health workers.
Early Diagnosis and Treatment
Early diagnosis and effective treatment can cure infection, prevent further morbidity and progression to severe disease and death, and arrest transmission. This intervention requires timely and accurate diagnosis; use of efficacious drugs; education of patients and their families about the disease, home management, and prevention; and referral to higher levels of the health system. The following are critical to the effectiveness of this intervention:
- Timeliness. A febrile malaria attack warrants early treatment. If left untreated, a proportion of P. falciparum malaria infections, perhaps 1 in 250 (and to a far lesser extent infections with other malaria species) will progress to severe disease within a few hours to a few days (Greenwood and others 2005). The globally agreed goal is that diagnosis and effective treatment should be provided within 24 hours of the onset of symptoms and signs.
- Diagnosis and effective drug treatment. An accurate diagnosis of malaria is based on detection of the parasite and, if laboratory diagnosis is not feasible, on clinical grounds. Health workers must monitor the therapeutic efficacy of drugs closely and change treatment policies when parasite resistance to chloroquine (figure 21.2), SP, and other drugs emerges (Baird 2005; Laxminarayan and others 2006; WHO 2002a). Concerns include unreliable and inaccurate microscopy and the disadvantages of alternative tests plus the widespread distribution and use of substandard and counterfeit drugs. The recommended treatments for malaria in areas with resistance to single drugs are combination treatments, preferably artemisinin combination therapy (ACT) (WHO 2001a, 2001b, 2003a, 2005). While ACT is a welcome, life-saving approach, more information on the cost-effectiveness of this new strategy is needed (Arrow, Panosian, and Gellband 2004; Yeung and others 2004). Baird (2005) reports that ACT costs range from US$2.00 (artesunate-amodiaquine, three doses in 48 hours) to US$9.12 (artemether-lumefantrine, six doses in 48 hours) per adult treatment; WHO has obtained the latter drug for US$2.40 per adult treatment for qualified purchasers, meaning those from low-income malarious countries.
- Location of clinical management. Effective management of patients requires skilled and well-equipped personnel at all levels of the health system. The two strategies for delivering antimalarials effectively are through health facilities and in or near the home when access to health facilities is limited. Given the pervasiveness of malaria infections, more information on the most effective and efficient ways to promote home treatment is urgently needed.

Figure 21.2
Chloroquine Treatment Failure in Africa, 1997–2002
Evidence for the effectiveness of early diagnosis and treatment is available from two different epidemiological conditions and health systems. In areas of low to moderate transmission, for example, southern Africa, the Americas, and Southeast Asia, where health care systems are relatively effective, the two major consequences of prompt and effective intervention are reduction of the period of infectivity of infected persons, and thus reduced transmission and incidence, and a lowered case-fatality rate and overall mortality. In Vietnam and the KwaZulu-Natal province of South Africa, P. falciparum malaria incidence and mortality rates fell when effective treatment policies (artesunate and ACT) replaced failing monotherapies (Hung and others 2002). The effective drug policies were implemented in conjunction with enhanced vector control; thus, effective treatment alone does not account for the fall in malaria incidence and mortality.
In areas with stable, high transmission of P. falciparum malaria—for instance, Papua New Guinea and Sub-Saharan Africa—where access to treatment is poor, little is known about the impact of early and effective treatment on malaria transmission. With a high EIR (10 to 1,000), a reduction in transmission intensity is unlikely to affect the incidence of disease until low EIRs (less than 10) are reached.
IPT in Pregnancy and Infancy
IPT is recommended in pregnancy in areas with high and stable transmission of P. falciparum malaria; IPT usually consists of two curative doses of antimalarial treatment. The recommended drug is SP given during the second and third trimesters of pregnancy during prenatal care visits. IPT in infancy involves giving infants treatment doses during vaccination or well-baby visits to health clinics.
In primigravid and secundigravid women, the incidence of severe maternal anemia, the incidence and density of placental parasitemia, and the incidence of low birthweight were 25 to 95 percent lower when mothers were given IPT than when they were not (Kayentao and others 2005; Steketee and others 2001). While initial studies indicate that IPT in infancy reduces anemia and febrile episodes, more research is needed. SP is becoming ineffective for IPT, and health experts are suggesting ACT as a replacement (White 2005).
Chemoprophylaxis
Chemoprophylaxis is advised by travel medicine specialists for nonresidents of endemic areas who are exposed to malaria for short periods. WHO does not recommend chemoprophylaxis for permanent residents of endemic areas because of low feasibility and compliance and high costs relative to the public health benefits (WHO 1996, 2000a, 2000b). The choice of chemoprophylaxis will depend on the drug-sensitivity profile, tolerance, side effects, costs, regimen, and compliance by patients (Baird 2005; Kain, Shanks, and Keystone 2001; White and Breman 2005). The effectiveness of chemoprophylaxis will depend mainly on patient compliance and parasite susceptibility.
Supervision and Policy Change
In relation to drug use, all control activities must be supervised and evaluated rigorously to ensure high-quality care for patients and program effectiveness and efficiency (Bryce and others 1994; Jha, Bangoura, and Ranson 1998). Studies in 37 countries indicate the need for a treatment policy change to ACT (WHO 2002a). Several countries in Africa, Asia, and South America are now implementing ACT as the first-line treatment for uncomplicated malaria (WHO 2005). Many countries are also trying to improve the time lag before treatment. More than 83 percent of the population in the three malarious provinces in South Africa is within 10 kilometers of a health facility (Sharp and le Sueur 1996). In Burkina Faso, Ethiopia, and Uganda, where access to clinics was poor and difficult, mothers and community health workers were empowered to dispense treatment, which resulted in major reductions in mortality and morbidity in children (Kidane and Morrow 2000; Pagnoni and others 1997; Sirima and others 2003).
Insecticide-treated Nets
The use of ITNs (bednets, curtains, and other materials) to provide personal protection by killing or repelling mosquitoes is one of the major strategies of malaria control (RBM 2002). Pyrethroids are recommended for the periodic treatment or retreatment of the protective materials.
The effectiveness of ITNs depends on their acceptability by the population at risk and their affordability. It is contingent on the habits, biology, and susceptibility of the mosquito vector; the compliance of the human population; and the concentration of insecticide on or in the fiber, which has to be maintained by regular re-treatment or by incorporating the insecticide in the fiber for long duration.
Over 20 studies in Africa and Asia have demonstrated more than 50 percent protective efficacy for individual users of ITNs in reducing malaria episodes, 29 percent protection against severe malarial disease, and substantial protection against anemia (Lengeler 2004). Most importantly, the use of ITNs reduced child mortality by 18 percent in five sites in Sub-Saharan Africa (Lengeler 2004).
Lengeler's (2004) review demonstrates the efficacy of ITNs in both stable and unstable transmission areas. Widespread use of ITNs resulted in an overall reduction in mortality of 19 percent, protected against anemia, and had a substantial impact on mild disease episodes. One large-scale rural study in Tanzania found that ITNs and untreated nets reduced mortality of children one month to four years, with protective efficacies of 27 and 19 percent, respectively (Armstrong-Schellenberg and others 2001). Re-treating ITNs semiannually or just before the annual peak in transmission is essential for effective vector control and is proving a major logistical and financial challenge. Fortunately, new types of nets with a long-lasting insecticidal property are now available, and retreatment will soon cease to be an issue. The salutary impact of large-scale ITN programs has been demonstrated in China (Tang 2000), Tanzania (Abdulla and others 2001; Armstrong-Schellenberg and others 2001; WHO 2005), and Vietnam (Hung and others 2002). More operational experience is required to inform national initiatives to scale up ITN use (Lengeler and Sharp 2003); yet, recent encouraging reports show that Eritrea, Malawi, Togo, Zambia, and other countries in Africa are already scaling up nationally with high coverage.
Vector Control
The reduction of Anopheles breeding and biting of humans involves different methods of insecticide and repellent application, environmental management, and behavioral change of populations at risk.
Indoor Residual Spraying of Dwellings with Insecticide
Indoor residual spraying (IRS) is the application of long-lasting insecticides (up to six months) on the walls of dwellings. Insecticides repel mosquitoes from entering houses or impart a lethal dose of the insecticide on the female mosquito when it rests on a sprayed surface, thereby preventing subsequent transmission. IRS is most effective against indoor-biting (endophilic) mosquito vectors. Vector susceptibility and post-feeding behavior are the main criteria to be considered when choosing an insecticide: organophosphates, carbamates, and pyrethroids are the main compounds used although some countries still rely on organochlorines (dichlorodiphenyl-trichloroethane, or DDT).
The effectiveness of this intervention depends on cost, toxicity, acceptability of the insecticide, its residual effects, and local political and international partnership commitment. Malaria incidence decreased sharply following the use of IRS in large-scale programs in many parts of Africa, the Americas, Asia, and Europe (Lengeler and Sharp 2003).
In southern Africa, more than 13 million people in six countries are protected from malaria (WHO 2002c). Control was initiated through the use of IRS and supported by engineering approaches, larviciding, prompt diagnosis, and effective treatment. Examples of successful and sustained malaria elimination using IRS with effective drug treatment are available from Cyprus, Greece, Portugal, Spain, and the former Yugoslavia and its successor states (Curtis and Mnzava 2000). The most successful malaria control efforts were linked closely to research and took place in many parts of Asia, where the notable example is the near eradication of malaria from Sri Lanka in the early 1960s, and in Central and South America from the late 1950s to early 1970s (Alilio, Bygbjerg, and Breman 2004; Carter and Mendis 2002; Gilles 2002).
Larviciding and Fogging
Larviciding is the application of chemical insecticides, including those of biological origin and insect growth regulators, to breeding sites. These may be applied to all mosquito breeding sites or targeted to the breeding sites of specific vectors. Recommended compounds, formulations, and dosages for larviciding are available through the WHO Pesticides Evaluation Scheme. Fogging or space spraying with insecticides requires specialized equipment, because the particle size of the insecticide determines its suspension qualities in the air, the number of droplets, and the penetration of space. The insecticide is not effective for as long as with IRS or ITNs, and application must occur during periods of peak target mosquito activity, generally at night.
Larviciding is not generally indicated for large-scale vector control in rural endemic areas because of the difficulty of locating all breeding sites, their often temporary nature, and the required frequency of application. Thus, larviciding is usually limited to urban areas, refugee camps, and industrial and development projects. Despite its impact on mosquito density and its contribution to reducing transmission, larviciding is not as effective as IRS and ITNs in reducing mosquito longevity (Najera and Zaim 2002).
Civil Engineering
Draining and filling larval breeding sites is one of the oldest methods of mosquito control and must be targeted to the breeding sites of locally important malaria vectors. Civil engineering strategies can require costly heavy equipment and materials and are useful for eliminating permanent breeding sites in urban areas, which are increasing globally, and at development project sites where earth removal has occurred.
Home Repellents and Insecticide Use
Commercially available mosquito repellents are applied directly on the skin or clothing as aerosols, lotions, or creams and contain active ingredients that protect the individual from mosquito bites. Commercially available mosquito coils containing pyrethroids can be burnt to repel mosquitoes, and electrically heated dispensers serve a similar function. Some communities in endemic regions use smoke, burning herbs, or plants to deter mosquitoes from entering the home.
N,N-diethyl-meta-toluamide (DEET) is the most widely used and effective ingredient in commercially available repellents (Curtis and others 1991). While several studies have shown that mosquito coils are effective at repelling mosquitoes, they are not as effective as ITNs (Charlwood and Jolly 1984).
Health Education and Counseling
Health education is the provision of information via newspapers, radio, or television, and health counseling is interactive, is individual, and involves the transfer of skills. The provision of information to households on ways to prevent malaria is needed in all endemic communities. It should cover the importance of early treatment and where to access it, the use of referral services, and the significance of full compliance with treatment and other interventions. The necessary information can be provided by community and voluntary health workers. These persons are an extension of the health system and work under the direct supervision of health facility staff or nongovernmental organizations and in conformity with standards and norms established by the national government (Gilles 2002). Such information can help to increase the standard of patient care and prevention programs by promoting citizen and community advocacy and demand for control.
Economics of Malaria Control Interventions
Goodman, Coleman, and Mills's (2000) study represents the most thorough attempt to compare the cost-effectiveness of a wide range of malaria control interventions. They find that in a very low-income country, the cost-effectiveness range per DALY averted was US$19 to US$85 for ITNs (nets plus insecticide), US$32 to US$58 for residual spraying (two rounds per year), US$3 to US$12 for chemoprophylaxis for children (assuming an existing delivery system), US$4 to US$29 for IPT for pregnant women, and US$1 to US$8 for case-management improvements. Goodman, Coleman, and Mills (2000) find that even though some interventions are relatively cheap, achieving high coverage may require a level of expenditure currently out of reach for many African countries and that overcoming operational barriers to achieving widespread coverage is likely to require substantial assistance from external donors.
Analysis
The following analysis incorporates new knowledge on the effects of interventions and on their costs for a low-income, Sub-Saharan African population living in an area of high, stable transmission. The modeling draws on a wide range of sources on the costs and effects of each intervention, extrapolated across settings and operational conditions. The approach allows for changing cost-effectiveness over time, for example, as resistance to antimalarial drugs or insecticides increases. To address problems of uncertainty in relation to many of the parameters, we used probabilistic sensitivity analysis, which allows for multivariate uncertainty by assigning ranges rather than point estimates to input variables. We assumed that cost and effectiveness input variables follow uniform triangular or normal continuous probability distributions (Mulligan, Morel, and Mills 2005).
We consider the cost-effectiveness of a limited subset of interventions: ITNs, IRS, IPT during pregnancy, and patient management with a change of the first-line drug. We include costs to the provider and the community and incremental out-of-pocket expenses for households, but because of major valuation and measurement problems, we do not include the indirect costs of patients' time to seek care and of the lost productivity resulting from morbidity. We consider only gross costs for all interventions except patient management, given the uncertainty inherent in estimating savings.
Insecticide-treated Nets
We based our analysis of ITNs on the delivery mechanism used in the WHO Special Programme for Research and Training in Tropical Diseases (WHO/TDR) trials, where householders, community health workers, and program staff worked together to treat the nets. In relation to insecticide, we considered permethrin and deltamethrin. Deltamethrin is effective for a year; thus, re-treatment is annual. Permethrin lasts for six months; thus, we assumed two treatments per year if the transmission season is longer than six months. The activities undertaken were the training of staff and community health workers, a campaign to inform the community about the intervention, the procurement and transport of the insecticide and nets, and the initial treatment and the re-treatment of the nets. We calculated cost-effectiveness for each intervention for two scenarios: one whereby nets were distributed to households and the second whereby treatment was arranged for existing nets. We drew estimates of the effectiveness of ITNS from a recent meta-analysis of WHO/TDR-sponsored trials conducted in Sub-Saharan Africa (Lengeler 2004). We adjusted the key parameter and effectiveness estimates to account for the proportion of children sleeping and not sleeping under a recently treated net.
With one treatment per year using deltamethrin, the mean cost per DALY averted was US$11 (90 percent range of US$5 to US$21). With one treatment of permethrin per year the cost-effectiveness ratio (CER) increased slightly to a mean of US$12 (90 percent range of US$6 to US$20). Two treatments of permethrin per year increased the mean CER to US$17 (90 percent range of US$9 to US$31). Even if net coverage is low and nets have to be distributed and treated, the intervention remains an extremely attractive use of resources. Moreover, the model includes health benefits only for children under five. If we included benefits for other household members, the CERs would be lower.
Insecticide Residual Spraying
We considered four insecticides for our analysis of the cost-effectiveness of IRS: DDT; malathion; and two pyrethroids, deltamethrin and lambda-cyhalothrin. We used the results of the Cochrane Review meta-analysis of ITN trials conducted in Africa to approximate the results of spraying on morbidity and mortality and adjusted effectiveness estimates to account for noncompliance (Lengeler 2004).
We found little difference between the CERs for the four insecticides when one round of spraying was done per year: they ranged from US$5 to US$18. With two rounds per year, costs increased, but we assumed that effectiveness remained the same, so all the CERs approximately doubled to US$11 to US$34. These results should be interpreted with caution because of uncertainty in relation to the estimates of effectiveness in children under five. The model was based on one round of spraying per year in areas of seasonal transmission and two rounds per year in areas of high, intense (perennial) transmission. Effectiveness will depend on the length of the transmission seasons and on the insecticide. DDT lasts for six months or more, lambda-cyhalothrin for three to six months, and malathion and deltamethrin for only two to three months (Lengeler 2004; Lengeler and Sharp 2003).
IPT during Pregnancy
We analyzed the cost-effectiveness of IPT assuming that primigravid women are given two or three doses of SP at a prenatal clinic. We analyzed benefits to the child by decreased mortality and benefits to the mother resulting from changes in the incidence of severe anemia. We estimated the effect of IPT on the neonatal mortality rate as a function of increased birthweight based on the Cochrane meta-analysis of malaria prevention in pregnancy (Gülmezoglu and Garner 1998). The model allowed for level of drug resistance, probability of initial attendance at a prenatal clinic, probability of returning for a second visit, probability of returning for a third visit, and compliance with the drug regimen. We estimated both incremental and average costs.
The incremental CER for IPT in pregnancy using SP had a 90 percent range from US$9 to US$21 with a mean of US$13. Average total cost-effectiveness had a mean of US$24 (90 percent range of US$16 to US$35).
Change in First-Line Drug
We analyzed the cost-effectiveness of changing first-line therapies to SP and to ACT using a patient-management model with a decision-tree framework in which a patient presents with uncomplicated malaria at an out-patient facility and progresses to full recovery, recovery with neurological sequelae, or death. Three potential drug policy changes were considered (see table 21.5). For current drug policies we assumed either chloroquine or SP as the first-line drug, with SP or amodiaquine as a second-line drug and quinine as the third-line drug. We then examined the cost-effectiveness of policy switches to either SP or ACT as first-line drug (with amodiaquine as second line and quinine as third line).
Table 21.5
Change in First-Line Drug.
Amodiaquine was chosen as the second-line drug in the new drug policy because, like chloroquine and SP, it is relatively cheap. Low compliance, adverse effects, and potential cross-resistance between amodiaquine and chloroquine excluded it from first-line selection. We omitted other potential drugs to limit the scope of analysis.
We used commonly found levels of drug resistance to create likely ranges. When used as second-line treatment, we assumed that a drug faced half the level of resistance than when it was used as a first-line drug. We estimated the growth rate of resistance to each drug based on its expected current location along a sigmoid growth curve. We assessed compliance with each drug based on the length and complexity of each regimen. We gave the widest range (20 to 70 percent) to compliance with ACT, which usually requires a three-day treatment, to account for the different lengths of regimen and variety of formulations.
Figure 21.3 shows that a switch from chloroquine to SP is cost-effective (less than $150 per DALY averted) when chloroquine resistance is above 35 percent. Switching from chloroquine to ACT becomes cost-effective as chloroquine resistance reaches around 37 percent. Switching from SP to ACT becomes cost-effective as SP resistance reaches 12 percent. This low threshold is due to the high growth rate of resistance to SP when it is used as a first-line therapy.
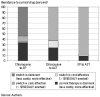
Figure 21.3
Cost-Effectiveness of Switching the First-Line Drug
Results and Interpretation
Table 21.6 presents the average CERs for the interventions reviewed. All interventions can be considered attractive using a cutoff of US$150 per DALY averted. For the childhood preventive interventions, the level of existing infrastructure is a key factor in influencing cost-effectiveness. ITNs are an even better use of resources if net coverage is already high, and IPT is even more cost-effective if prenatal care coverage is good. However, even if levels of infrastructure are poor, these interventions are still attractive based on cost-effectiveness criteria.
Table 21.6
CERs for ITNs, IRS, and IPT (2001 US$).
Curtis and Mnzava's (2000) review comparing worldwide trials of ITNs and IRS for malaria control suggests that they were of equivalent effectiveness. Lengeler and Sharp (2003 21,) also conclude that choosing between IRS and ITNs is "largely a matter of operational feasibility and availability of local resources, rather than one of malaria epidemiology or cost-effectiveness." DDT is the cheapest insecticide but is seldom used because of concerns about its environmental impact. An updated systematic review of the health effects of IRS as well as a full economic comparison between spraying with DDT and other insecticides and ITNs is urgently required. Such reviews should include the environmental benefits of reducing DDT levels and the costs of alternative interventions.
As effective patient management, IPT, and other approaches toward drug use become more widespread, drug resistance will increase, which will affect cost-effectiveness. In terms of first-line treatment, while a switch from chloroquine to SP is unlikely to be costly, cost-effectiveness depends on the initial level of severe resistance to each drug. A switch from chloroquine to ACT is likely to be costly but more effective, given that resistance to ACT is essentially nonexistent and the growth rate of resistance to ACT is likely to be low (Yeung and others 2004). Given the high growth rate of resistance to SP, switching to ACT becomes cost-effective when SP resistance surpasses 12 percent, a relatively low threshold. A switch from chloroquine to ACT appears to be highly cost-effective at all initial levels of chloroquine resistance above 37 percent. Recent studies indicate the remarkable effectiveness of the ACT artemether-lumefantrine in East African areas of chloroquine, amodiaquine, and SP resistance (Mutabingwa and others 2005; Piola and others 2005); it is expected that the availability of ACTs will increase and the costs will decrease in the near future.
Affordability and Scaling Up
Cost-effectiveness analyses can identify which interventions are the most efficient to implement, but information is also needed on affordability. Some interventions are cheap, such as prevention in pregnancy. Achievement of high coverage with an intervention to prevent childhood malaria (for example, ITNs) has a high total cost, which needs to be borne in part by external funding. For example, full coverage of children (assuming 22 percent of the population is under five years of age) would cost US$2.81 million per 1 million persons of the general population covered (assuming the provision of nets plus two rounds of permethrin). The same coverage with IRS would cost about US$4.01 million using deltamethrin (two rounds).
In addition to the difficulties of financial feasibility, the implementation of ITN interventions poses operational and logistical challenges. A number of strategic approaches are available for national ITN upscaling (RBM 2002). The main ones are social marketing (for example, in Malawi and Kenya), assisted commercial sector development (Senegal, Mali, Tanzania), and totally free distribution (Togo). An important feature of all the approaches is that their cost per net distributed decreases significantly as the scale of the undertaking increases, so it is hard to use available estimates to project cost in national programs.
Independent of the main ITN distribution strategy, a number of countries are implementing additional actions targeted at the main high-risk groups. For example, a national voucher scheme is currently being implemented in Tanzania to provide every pregnant woman in the country with a free ITN, and a net is being distributed to children at the time of measles vaccination in Togo.
Re-treatment of ITNs on a large-scale remains a formidable operational issue, and free distribution of insecticide in the way it is done in Vietnam and China is probably the best way forward. This should occur while waiting the availability of long-lasting insecticidal nets that do not require retreatment.
Curtis and Maxwell (2002) point to the successful experience of Vietnam, where ITNs now protect 10 million people and the public health service provides free insecticide. This approach in Africa would require a substantial commitment by donors and governments given that most African governments spend only around US$4 per capita per year on health (World Bank 2003). Eritrea, with 65 percent ITN coverage, and other African countries are beginning to make great strides in getting ITNs to their populations (Barat 2006; WHO 2005; World Bank 2005).
The move toward ACT poses the most difficult questions in relation to the long-term affordability of malaria control interventions. After scale-up, estimates indicate that the additional annual costs of ACT versus current failing drugs range from US$300 million to US$500 million globally (Arrow, Panosian, and Gellband 2004), with the precise amount depending on the extent to which all fevers are treated. This figure does not include drugs for nonmalarial fevers in endemic areas and the costs for the substantial health system strengthening required to make the most effective use of ACT. These include the costs of improved drug regulation, pharmacovigilance, diagnostics, and implementation of different drug policies for different population groups. The introduction of ACT has permanently changed the economic landscape of malaria control. Innovative funding solutions at the global level are required to ensure that effective drugs are made available to the most vulnerable groups. The Global Fund to Fight AIDS, Tuberculosis, and Malaria is providing the major leadership and resources for securing ACTs and other commodities, and many other agencies and organizations are joining coalitions to combat malaria through control, research, training, funding, and advocacy activities (Breman, Alilio, and Mills 2004; Feacham 2004: http://www.theglobalfund.org/en).
Economic Benefits of Malaria Control
Given the substantial total costs of several malaria control interventions, the case for introducing them can be strengthened by evidence on non-health-related benefits, especially evidence of income gains or prevention of income losses. Three different approaches to measuring such benefits are assessing the direct and indirect costs of malaria, studying the relationship between malaria and the output of agricultural or industrial activities, and exploring the macroeconomic impact of malaria. Only the third approach sheds much light on the benefits of control as opposed to the burden of the disease in the absence of control. This point is important, because in places facing the most severe malaria problems, direct information on the economic benefits of control is largely unavailable.
Direct and Indirect Costs of Malaria
The standard approach has been to view the two key determinants of the economic costs of malaria as the direct costs of expenditure on prevention and treatment and the indirect costs of productive labor time lost because of malaria morbidity and mortality, and to estimate the total economic impact by adding the direct and indirect costs (Mills 1992). Households use a range of preventive measures (mosquito coils, aerosol sprays, bednets, and mosquito repellents) to differing degrees. A review of evidence for Sub-Saharan Africa found that monthly per capita household expenditures ranged from US$0.05 per person in rural Malawi to US$2.10 in urban Cameroon, equivalent to US$0.24 and US$15 per household in 1999 U.S. dollars (Chima, Goodman, and Mills 2003). The costs of treatment for malaria include out-of-pocket expenditures for consultation fees, drugs, transport, and subsistence at a distant health facility. For Sub-Saharan Africa, these costs ranged between US$0.41 and US$3.88 per person, equivalent to between US$1.88 and US$26 per household per year. Household expenditure on treatment is usually highly regressive, consuming a much larger proportion of income in the poorest households.
Computations of public expenditures on malaria prevention and treatment are imprecise because most fall within general health service expenditures. About 20 to 40 percent of outpatient visits in malarious Africa are for fever, and suspected malaria among inpatients ranges from 0.5 to 50.0 percent of admissions. Kirigia and others (1998) found that inpatient treatment for pediatric malaria absorbed 15 percent of the annual recurrent costs of inpatient care in one Kenyan hospital and 9 percent in another. Ettling and Shepard (1991) estimated that Rwanda's Ministry of Health spent 19 percent of its operating budget on malaria treatment. Because of the dominance of out-of-pocket spending by households, public expenditures on malaria generally account for a minority of total malaria expenditure.
The methods used to measure and value time lost and a day's work vary considerably between studies; the average time lost per episode for a sick adult and for an adult caring for sick children ranged from one to five days. The average indirect cost per episode ranged from US$0.68 for children under 10 years of age in Malawi to US$23 per adult episode in Ethiopia. Authors have concluded that aggregate productivity losses can be significant for households and for the economy as a whole. In Malawi, the indirect costs of malaria were equivalent to 2.6 percent of annual household income (Ettling and others 1994), and Russell (2004) reports that malaria generally consumes less than 10 percent of family income. Leighton and Foster (1993) estimate that the total annual value of malaria-related production losses was 2 to 6 percent of gross domestic product in Kenya and 1 to 5 percent in Nigeria. The productivity consequences of mortality have received relatively little attention.
The direct and indirect cost approach involves two methodological problems. First, calculations are based mainly on days of illness and neglect mortality, debility (usually from anemia), and neurological and other long-term sequelae. Second, households' coping strategies are likely to reduce the immediate impact of illness, although in the long term they may impose costs through the sale of assets such as livestock, which jeopardizes a household's asset base.
Relationship between Malaria and Output
With the exception of a study of cotton production in Côte d'Ivoire, where the prevalence of parasitemia above a cutoff density had a major impact on labor efficiency (Audibert, Mathonnat, and Henry 2003), studies have not succeeded in identifying impacts on output. One possibility is that the risk of malaria may discourage the planting of crops that require intensive cultivation, the settlement and cultivation of fertile land, or the development of tourism and industry in suitable locations, but good evidence is lacking.
Macroeconomic Impact of Malaria
Recent empirical cross-country comparisons of economic growth indicate that eliminating malaria would have a strong positive impact on economic development. Gallup and Sachs (2001) use cross-country regression analysis to relate the growth in gross domestic product per capita between 1965 and 1990 to initial income levels, initial human capital stock, policy variables, geographical variables, and a malaria index calculated as the product of the fraction of land area with endemic malaria in 1965 and the fraction of malaria cases that were due to P. falciparum in 1990. Their results suggest that countries with a substantial amount of malaria grew 1.3 percent per year less than countries with little or no malaria between 1965 and 1990 (controlling for other influences on growth) and that a 10 percent reduction in malaria was associated with 0.3 percent higher growth per year. McCarthy, Wolf, and Wu (2000) employ a similar approach to explore the impact of malaria on average per capita growth rates during three five-year periods. They find a significant negative association between malaria and economic growth, although the estimated impact differed sharply across countries. The impact was smaller than that found by Gallup and Sachs (2001), exceeding 0.25 percent per year in only a quarter of the sample countries and averaging 0.55 percent for those in Sub-Saharan Africa.
Benefit–Cost Ratios
Mills and Shillcutt 2004 related the evidence on the macroeconomic benefits of malaria control to information on the costs of reducing malaria to calculate a benefit-cost ratio (Mills and Shillcutt 2004). Depending on the assumptions, the benefit-cost ratio ranged between 1.9 and 4.7 (using a 3 percent discount rate). In terms of economic growth alone malaria control is extremely cost beneficial.
Research Priorities
Current interventions to combat malaria remain inadequate for achieving the increased levels of successful patient management and prevention to which all malarious countries aspire and for which ambitious targets have been set (box 21.1). Greatly increased support for malaria research and for developing institutional capacity must occur to make advances and to bring them to populations in need. The WHO Scientific Working Group on Malaria and others have identified four major areas of research as follows (Remme and others 2002; WHO 1996; WHO 2003c).
Patient Management
Patient management, including treatment, should address the following:
- evaluation of treatment effectiveness and of access to treatment for uncomplicated malaria in children and during pregnancy, with an emphasis on home management and evaluation of alternative delivery systems
- investigation of the pathogenesis of malaria, in particular anemia and immune response mechanisms
- evaluation of new approaches, for example, rectal drug administration, for managing severe illness
- evaluation of ACT, including delivery approaches via the public and private sectors
- development of new drugs with novel targets; the Medicines for Malaria Ventura (MMV) is spearheading this activity.
Prevention Research
Prevention research should focus on the following:
- new approaches to drug-based malaria prevention, including IPT in children and during pregnancy
- strategies for scaling up the use of ITNs.
Innovative Approaches
Innovative approaches should use new technologies, including recent advances in sequencing the DNA of P. falciparum and A. gambiae, to achieve the following:
- discover and develop drugs, diagnostics, vaccines, insecticides, and antiparasite effector molecules using genomics
- carry out strategic and basic research on vector-parasite-host interactions
- assess mechanisms for addressing drug and insecticide resistance
- develop and carry out field evaluations of transgenic methods for interrupting malaria transmission.
Policy Research
Social, economic, and policy research should focus on the following:
- developing and applying a common methodology for measuring socioeconomic status
- carrying out policy and operational research on the impact, viability, sustainability, and optimal balance of public-private partnerships
- investigating ethical, legal, and social issues pertaining to new malaria-related tools.
Capacity strengthening for research and operations (including clinical trials) is urgently needed. This strengthening will result in the ability to better evaluate new drugs and vaccines and existing malaria control tools (ITNs, IPT) and to tackle the scaling up of malaria strategies.
A classification of research priorities by time frame includes the three-year, five-year, and ten-year targeting.
Three-year completion targeting includes the following:
- apply to the U.S. Food and Drug Administration (FDA) with evidence for two separate fixed-dose artemisinin combinations at a target adult treatment price of US$1.00, or US$0.60 or less for children; two combinations are necessary, as one may fail in testing or have other unforeseen problems
- evaluate the two best candidate ACT drugs for IPT in pregnant women and in infancy and early childhood (IPTi)
- launch studies to reach an evidence-based conclusion on the costs and benefits of long-lasting ITNs versus IRS in Sub-Saharan Africa and other endemic areas
- carry out operational studies to determine the best methods of deploying ACT through the public and private sectors so that first-level patient management can occur at the home and at the village levels; this involves studying packaging and distribution networks, assessing adherence, and addressing how to deploy and supervise use of artesunate rectal suppositories
- conduct economic reviews and predictive modeling to make an economic case for increased international investment in malaria control, including collecting detailed data from several scaled-up, national, fully supported control programs employing the best available strategies and interventions
- conduct in Africa and other endemic continents a maximum number of FDA-compliant phase I and phase II malaria vaccine trials to select candidates for clinical trials.
The five-year completion target is as follows:
- develop and deploy more sensitive, specific, and predictive diagnostic tests that are inexpensive and practical
- carry out operational studies on how to deploy diagnostics in areas of low, intermediate, and high transmission.
The ten-year completion target has these focuses:
- develop an inexpensive, safe, and synthetic trioxane anti-malarial drug
- develop another new drug with a target and resistance mechanism that is unrelated to existing drugs
- carry out basic insecticide research to develop new approaches to both personal protection and residual house spraying
- launch successful phase III and phase IV field testing of malaria vaccines to prevent clinical illness and transmission with licensure by regulatory bodies
- use genomic, proteomic, and bioinformatic tools to better understand the pathogenesis of malaria, to design new drugs and vaccine candidates for training scientists, and to transfer technologies
- train a critical mass of leaders in science and operations to carry out the required research in support of control.
Conclusion
Given the heavy burden of malaria, the need to use existing strategies and interventions in scaled-up programs more effectively and to deploy them more widely is urgent and merits the highest priority, especially in Africa. While existing tools can be improved, newer tools are required. The history of malaria research and control shows that they are synergistic. Integrating research and control activities has resulted in success in several areas of the world, and will result in vanquishing malaria early in the 21st century.
References
- Abdulla S., Schellenberg J. A., Nathan R., Mukasa O., Marchant T., Smith T. et al. Impact on Malaria Morbidity of a Programme Supplying Insecticide Treated Nets in Children Age under Two Years in Tanzania: Community Cross-Sectional Study. British Medical Journal. 2001;322:270–73. [PMC free article: PMC26579] [PubMed: 11157527]
- Alilio M. S., Bygbjerg I., Breman J. G. Are Multilateral Malaria Research and Control Programs the Most Successful? Lessons from the Past 100 Years. American Journal of Tropical Medicine and Hygiene. 2004;70(Suppl. 2):268–78. [PubMed: 15331847]
- Alonso P. L., Sacarlal J., Aponte J. J., Leach A., Macete E., Milman J. et al. Efficacy of the RTS,S/AS02A Vaccine against Plasmodium falciparum Infection and Disease in Young African Children: Randomised Controlled Trial. Lancet. 2004;364:1411–20. [PubMed: 15488216]
- Armstrong-Schellenberg J. R., Abdulla S., Nathan R., Mukasa O., Marchant T. J., Kikumbih N. et al. Effect of Large-Scale Social Marketing of Insecticide-Treated Nets on Child Survival in Rural Tanzania. Lancet. 2001;357:1241–47. [PubMed: 11418148]
- Arrow, K. J., C. B. Panosian, and H. Gellband, eds. 2004. Saving Lives, Buying Time: Economics of Malaria Drugs in an Age of Resistance. Washington, DC: National Academy Press for Institute of Medicine. [PubMed: 25009879]
- Audibert M., Mathonnat J., Henry M. C. Malaria and Property Accumulation in Rice Production Systems in the Savannah Zone of Côte d'Ivoire. Tropical Medicine and International Health. 2003;8(5):471–83. [PubMed: 12753643]
- Baird J. K. Effectiveness of Antimalarial Drugs. New England Journal of Medicine. 2005;352:1565–77. [PubMed: 15829537]
- Ballou R., Arevalo-Herrera M., Carucci D., Richie T. L., Corradin G., Diggs C. et al. Update on the Clinical Development of Candidate Malaria Vaccines. American Journal of Tropical Medicine and Hygiene. 2004;71(Suppl. 2):239–47. [PubMed: 15331843]
- Barat L. M. Four Malaria Success Stories: How Malaria Burden Was Successfully Reduced in Brazil, Eritrea, India, and Vietnam. American Journal of Tropical Medicine and Hygiene. 2006;74(1):12–16. [PubMed: 16407339]
- Breman J. G. The Ears of the Hippopotamus: Manifestations, Determinants, and Estimates of the Malaria Burden. American Journal of Tropical Medicine and Hygiene. 2001;64(Suppl. 12):1–11. [PubMed: 11425172]
- Breman J. G., Alilio M. S., Mills A. Conquering the Intolerable Burden of Malaria: What's New, What's Needed: A Summary. American Journal of Tropical Medicine and Hygiene. 2004;71(Suppl. 2):1–15. [PubMed: 15331814]
- Bryce J., Boschi-Pinto C., Shibuya K., Black R. E. the WHO Child Health Epidemiology Reference Group. WHO Estimates of the Causes of Deaths of Children. Lancet. 2005;365(9465):1114–6. [PubMed: 15794969]
- Bryce J., Roungou J. B., Nguyen-Dinh P., Naimoli J. F., Breman J. G. Evaluation of National Malaria Control Programmes in Africa. Bulletin of the World Health Organization. 1994;72(3):371–81. [PMC free article: PMC2486696] [PubMed: 8062394]
- Carter R., Mendis K. Evolutionary and Historical Aspects of the Burden of Malaria. Clinical Microbiological Reviews. 2002;15(4):564–94. [PMC free article: PMC126857] [PubMed: 12364370]
- Caulfield L., Richard S. A., Black R. Undernutrition as an Underlying Cause of Malaria Morbidity and Mortality. American Journal of Tropical Medicine and Hygiene. 2004;71(Suppl. 2):55–63. [PubMed: 15331819]
- Charlwood D., Jolly D. The Coil Works against Mosquitoes in Papua, New Guinea. Transactions of the Royal Society of Tropical Medicine and Hygiene. 1984;78:678. [PubMed: 6506156]
- Chima R., Goodman C., Mills A. The Economic Impact of Malaria in Africa: A Critical Review of the Evidence. Health Policy. 2003;63(1):17–36. [PubMed: 12468115]
- Colebunders R., Ryder R., Francis H., Nekwei W., Bahwe Y., Lebughe I. et al. Seroconversion Rate, Mortality, and Clinical Manifestations Associated with the Receipt of a Human Immunodeficiency Virus-Infected Blood Transfusion in Kinshasa, Zaire. Journal of Infectious Diseases. 1991;164(3):450–56. [PubMed: 1869835]
- Craig M. H., Snow R. W., le Sueur D. A Climate-Based Distribution Model of Malaria Transmission in Sub-Saharan Africa. Parasitology Today. 1999;15:105–11. [PubMed: 10322323]
- Curtis, C. F., J. D. Lines, B. Lu, and A. Renz. 1991. "Natural and Synthetic Repellents." In Control of Disease Vectors in the Community, ed. C. F. Curtis, 75–92. London: Wolfe.
- Curtis C. F., Maxwell C. Free Insecticide for Nets Is Cost-Effective. Trends in Parasitology. 2002;18:204–5. [PubMed: 11983598]
- Curtis C. F., Mnzava A. E. P. Comparison of House Spraying and Insecticide-Treated Nets for Malaria Control. Bulletin of the World Health Organization. 2000;78(12):1389–1401. [PMC free article: PMC2560652] [PubMed: 11196486]
- Djimdé A. A., Dolo A., Quattara A., Diakité S., Plowe C. V., Doumbo O. K. Molecular Diagnosis of Resistance to Antimalarial Drugs during Epidemics and in War Zones. Journal of Infectious Diseases. 2004;190(4):853–55. [PubMed: 15272415]
- Ettling M. B., McFarland D. A., Schultz L. J., Chitsulo L. Economic Impact of Malaria in Malawian Households. Tropical Medicine and Parasitology. 1994;45:74–79. [PubMed: 8066390]
- Ettling M. B., Shepard D. S. Economic Cost of Malaria in Rwanda. Tropical Medicine and Parasitology. 1991;42(3):214–18. [PubMed: 1801149]
- Feacham R. G. A. The Research Imperative: Fighting AIDS, TB, and Malaria. Tropical Medicine and International Health. 2004;9(11):1139–41. [PubMed: 15548308]
- Fernando D., Wickremasinghe R., Mendis K. N., Wickremasinghe A. R. Cognitive Performance at School Entry of Children Living in Malaria-Endemic Areas of Sri Lanka. Transactions of the Royal Society of Tropical Medicine and Hygiene. 2003;97(3):161–65. [PubMed: 14584369]
- French N., Nakiyingi J., Lugada E., Watera C., Whitworth J. A., Gilks C. F. Increasing Rates of Malarial Fever with Deteriorating Immune Status in HIV-1 Infected Ugandan Adults. AIDS. 2001;15(7):899–906. [PubMed: 11399962]
- Gallup J. L., Sachs J. D. The Economic Burden of Malaria. American Journal of Tropical Medicine and Hygiene. 2001;64(Suppl. 1):85–96. [PubMed: 11425181]
- Gilles, H. M., ed. 2002. "Historical Outline." In Essential Malariology, 4th ed., ed. D. A. Warrell and H. M. Gilles, 1–7. New York: Arnold.
- Goodman, C., P. Coleman, and A. Mills. 2000. Economic Analysis of Malaria Control in Sub-Saharan Africa. Geneva: Global Forum for Health Research.
- Greenberg, A. E. 1992. "HIV and Malaria." In AIDS in the World, ed. J. M. Mann, D. J. Tarantola, and T. W. Netter, 143–48. Cambridge, MA: Harvard University Press.
- Greenberg A. E., Nguyen-Dinh P., Mann J. M., Kabote N., Colebunders R. L., Francis H. et al. The Association Between Malaria, Blood Transfusions, and HIV Seropositivity in a Pediatric Population in Kinshasa, Zaire. Journal of the American Medical Association. 1988;259:545–49. [PubMed: 3275815]
- Greenberg A. E., Ntumbanzondo M., Ntula N., Mawa L., Howell J., Davachi F. Hospital-Based Surveillance of Malaria-Related Paediatric Morbidity and Mortality in Kinshasa, Zaire. Bulletin of the World Health Organization. 1989;67(2):189–96. [PMC free article: PMC2491235] [PubMed: 2743538]
- Greenwood B. M., Bojang K., Whitty C. J. M., Targett G. A. T. Malaria. Lancet. 2005;365(9469):1487–98. [PubMed: 15850634]
- Gülmezoglu, A. M., and P. Garner. 1998. "Malaria in Pregnancy in Endemic Areas (Cochrane Review)." Cochrane Library 3, Oxford, Update Software.
- Hay S. I., Rogers D. J., Toomer J. F., Snow R. W. Annual Plasmodium falciparum Entomological Inoculation Rates (EIR) across Africa: Literature Survey, Internet Access, and Review. Transactions of the Royal Society of Tropical Medicine and Hygiene. 2004;94:113–27. [PMC free article: PMC3204456] [PubMed: 10897348]
- Holding P. A., Kitsao-Wekulo P. K. Describing the Burden of Malaria on Child Development: What Should We Be Measuring and How Should We Be Measuring It? American Journal of Tropical Medicine and Hygiene. 2004;71(Suppl. 2):71–79. [PubMed: 15331821]
- Hung I. Q., Vries P. J., Giao P. T., Nam N. V., Binh T. Q., Chong M. T. et al. Control of Malaria: A Successful Experience from Viet Nam. Bulletin of the World Health Organization. 2002;80(8):660–66. [PMC free article: PMC2567582] [PubMed: 12219158]
- INDEPTH (International Network of Field Sites with Continuous Demographic Evaluation of Populations and Their Health in Developing Countries). 2002. Population, Health, and Survival at INDEPTH Sites. Vol. 1 of Population and Health in Developing Countries. Ottawa: International Development Research Centre.
- Jha P., Bangoura O., Ranson R. The Cost-Effectiveness of Forty Health Interventions in Guinea. Health Policy and Planning. 1998;13(3):249–62. [PubMed: 10187595]
- Kain K. C., Shanks G. D., Keystone J. S. Malaria Chemoprophylaxis in the Age of Drug Resistance: I. Currently Recommended Drug Regimens. Clinical Infectious Diseases. 2001;33(2):226–34. [PubMed: 11418883]
- Kayentao K., Kodio M., Newman R. D., Maiga H., Doumtabe D., Ongoiba A. et al. Comparison of Intermittent Preventive Treatment with Chemoprophylaxis for the Prevention of Malaria during Pregnancy in Mali. Journal of Infectious Diseases. 2005;191(1):109–16. [PubMed: 15593011]
- Keiser J., Utzinger J., de Castro M. Caldas, Smith T. A., Tanner M., Singer B. H. Urbanization in Sub-Saharan Africa and Malaria Control. American Journal of Tropical Medicine and Hygiene. 2004;71(Suppl. 2):118–27. [PubMed: 15331827]
- Kidane G., Morrow R. H. Teaching Mothers to Provide Home Treatment of Malaria in Tigray, Ethiopia: A Randomised Trial. Lancet. 2000;356(9229):550–55. [PubMed: 10950232]
- Kirigia J. M., Snow R. W., Fox-Rushby J., Mills A. The Cost of Treating Pediatric Malaria Admissions and the Potential Impact of Insecticide-Treated Mosquito Nets on Hospital Expenditure. Tropical Medicine and International Health. 1998;3:145–50. [PubMed: 9537277]
- Korenromp E. L., Williams B. G., Gouws E., Dye C., Snow R. W. Measuring Trends in Childhood Malaria Mortality in Africa: A New Assessment of Progress toward Targets Based on Verbal Autopsy. Lancet Infectious Diseases. 2003;3:349–58. [PubMed: 12781507]
- Laxminarayan, R., Z. Bhutta, A. Duse, P. Jenkins, T. O'Brien, I. N. Okeke, A. Pablo-Mendez, K. P. Klugman. 2006. "Drug Resistance." In D. T. Jamison, J. G. Breman, A. Measham, and others, Disease Control Priorities in Developing Countries, eds. 2nd. ed. New York: Oxford University Press, ch. 55.
- Leighton, C., and R. Foster. 1993. "Economic Impacts of Malaria in Kenya and Nigeria." Abt Associates, Health Financing and Sustainability Project, Bethesda, Maryland.
- Lengeler, C. 2004. "Insecticide-Treated Bed Nets and Curtains for Preventing Malaria." Cochrane Database Systematic Reviews (2) CD000363. [PubMed: 15106149]
- Lengeler, C., and B. Sharp. 2003. "Indoor Residual Spraying and Insecticide-Treated Nets." In Reducing Malaria's Burden. Evidence of Effectiveness for Decision Makers. Washington DC: Global Health Council, 17–24. http://www
.globalhealth.org. - McCarthy, F. D., H. Wolf, and Y. Wu. 2000. "The Growth Costs of Malaria." In NBER Working Paper 7541, National Bureau of Economic Research, Cambridge, MA.
- Mendis K., Sina B. J., Marchesini P., Carter R. The Neglected Burden of Plasmodium vivax Malaria. American Journal of Tropical Medicine and Hygiene. 2001;64(Suppl. 1):97–105. [PubMed: 11425182]
- Menendez C., Fleming A. F., Alonso P. L. Malaria-Related Anemia. Parasitology Today. 2000;16:469–76. [PubMed: 11063857]
- Mills A. The Economic Evaluation of Malaria Control Technologies: The Case of Nepal. Social Science and Medicine. 1992;34:965–72. [PubMed: 1631609]
- Mills, A., and S. Shillcutt. 2004. "The Challenge of Communicable Disease." In Global Crises, Global Solutions, ed. B. Lomborg. Cambridge, U.K.: Cambridge University Press.
- Mulligan, J., C. Morel, and A. Mills. 2005. "Cost-Effectiveness of Malaria Control Interventions." Disease Control Priorities Project Background Paper, Disease Control Priorities Project, Bethesda, MD.
- Mung'Ala-Odera V., Snow R. W., Newton C. R. J. C. The Burden of the Neurocognitive Impairment Associated with Falciparum Malaria in Sub-Saharan Africa. American Journal of Tropical Medicine and Hygiene. 2004;71(Suppl. 2):64–70. [PubMed: 15331820]
- Murphy S. C., Breman J. G. Gaps in the Childhood Malaria Burden in Africa: Cerebral Malaria, Neurologic Sequelae, Anemia, Respiratory Distress, Hypoglycemia, and Complications of Pregnancy. American Journal of Tropical Medicine and Hygiene. 2001;64(Suppl. 1):57–56. [PubMed: 11425178]
- Murray, C. J. L., and A. D. Lopez. 1996. The Global Burden of Disease: A Comprehensive Assessment of Mortality and Disability from Diseases, Injuries, and Risk Factors in 1990 and Projected to 2020. Cambridge, MA: Harvard University Press.
- ———.1997Mortality by Cause for Eight Regions of the World: Global Burden of Disease Study Lancet 3491269–76. [PubMed: 9142060]
- Mutabingwa T. K., Anthony D., Heller A., Hallett R., Ahmed J., Drakeley C. et al. Amodiaquine Aone, Amodiaquine+Sulfadoxine-Pyrimethamine, Amodiaquine+Artesunate, and Artemether-Lumefantrine for Outpatient Treatment of Malaria in Tanzanian Children: a Four-Armed Randomized Effectiveness Trial. Lancet. 2005;365(9469):1474–80. [PubMed: 15850631]
- Najera, J. A., and M. Zaim. 2002. Malaria Vector Control: Decision Making Criteria and Procedures for Judicious Use of Insecticides. WHO/CDS/WHOPES/2002.5. Geneva: World Health Organization.
- Newman R. D., Parise M. E., Slutsker L., Nahlen B., Steketee R. W. Safety, Efficacy, and Determinants of Effectiveness of Antimalarial Drugs during Pregnancy: Implications for Prevention Programs in Plasmodium falciparum–Endemic Sub-Saharan Africa. Tropical Medicine and International Health. 2003;6:488–506. [PubMed: 12791054]
- Nosten F., McGready R., Simpson J. A., Thwai K. L., Balkan S., Cho T. et al. Effects of Plasmodium vivax Malaria in Pregnancy. Lancet. 1999;354(9178):546–49. [PubMed: 10470698]
- Pagnoni F., Convelbo N., Tiendrebeogo J., Cousens S., Esposito F. A Community-Based Programme to Provide Prompt and Adequate Treatment of Presumptive Malaria in Children. Transactions of the Royal Society of Tropical Medicine and Hygiene. 1997;91(5):512–17. [PubMed: 9463653]
- Piola P., Fagg C., Bajunirwe F., Biraro S., Grandesso F., Ruzagira E. et al. Supervised Versus Unsupervised Intake of Six-Dose Artemether-Lumefantrine for Treatment of Acute, Uncomplicated Plasmodium falciparum Malaria in Mbarara, Uganda: A Randomized Trial. Lancet. 2005;365(9469):1467–73. [PubMed: 15850630]
- RBM (Roll Back Malaria). 2002. Scaling-up Insecticide-Treated Netting Programmes in Africa: A Strategic Framework for Coordinated National Action. Geneva: WHO/CDS/RBM/2002.
- Remme J. H., Blas E., Chitsulo L., Desjeux P. M., Engers H. D., Kanyok T. P. et al. Strategic Emphasis for Tropical Diseases Research: A TDR Perspective. Trends in Parasitology. 2002;18(10):421–26. [PubMed: 12377584]
- Rosen J. B., Breman J. G. Malaria Intermittent Preventive Treatment in Infants (ITPi), Chemoprophylaxis, and Childhood Vaccinations. Lancet. 2004;363(9418):1386–88. [PubMed: 15110499]
- Russell S. The Economic Burden of Illness for Households in Developing Countries: Catastrophic or Manageable? A Review of Studies Focusing on Malaria, TB, and HIV/AIDS. American Journal of Tropical Medicine and Hygiene. 2004;71(Suppl. 2):147–55. [PubMed: 15331831]
- Savarit D., De Cock K. M., Shutz R., Konate S., Lackritz E., Bondurand A. Risk of HIV Infection from Transfusion with Blood Negative for HIV Antibodies in a West African City. British Medical Journal. 1992;305(6852):498–502. [PMC free article: PMC1882849] [PubMed: 1327367]
- Schellenberg D. S., Menendez C., Aponte J. J., Kahigwa E., Tanner M., Mshinda H., Alonso P. Intermittent Preventive Treatment for Tanzanian Infants: Follow-up to Age 2 Years of a Randomized Placebo-Controlled Trial. Lancet. 2005;365(9469):1481–83. [PubMed: 15850632]
- Sharp B. L., le Sueur D. Malaria in South Africa—The Past, the Present, and Selected Implications for the Future. South African Medical Journal. 1996;86(1):83–89. [PubMed: 8685790]
- Sirima S. B., Konaté A., Tiono A. B., Convelho N., Cousins S., Pagnoni F. Early Treatment of Childhood Fevers with Pre-packaged Antimalarial Drugs in the Home Reduces Severe Malaria Morbidity in Burkina Faso. Tropical Medicine and International Health. 2003;8(2):1–7. [PubMed: 12581438]
- Snow R. W., Craig M. H., Deichmann U., Marsh K. Estimating Mortality, Morbidity, and Disability Due to Malaria among Africa's Non-pregnant Population. Bulletin of the World Health Organization. 1999;77:624–40. [PMC free article: PMC2557714] [PubMed: 10516785]
- Snow, R. W., M. H. Craig, C. R. J. C. Newton, and R. W. Steketee. 2003. "The Public Health Burden of Plasmodium falciparum Malaria in Africa: Deriving the Numbers." Working Paper 11, Disease Control Priorities Project, Bethesda, MD.
- Snow R. W., Guerra C. A., Noor A. M., Myint H. Y., Hay S. I. The Global Distribution of Clinical Episodes of Plasmodium falciparum Malaria. Nature. 2005;434:214–17. [PMC free article: PMC3128492] [PubMed: 15759000]
- Snow R. W., Korenromp E., Drakely C., Gouws E. Pediatric Mortality in Africa: Plasmodium falciparum Malaria as a Cause or Risk? American Journal of Tropical Medicine and Hygiene. 2004;70(Suppl. 2):16–24. [PubMed: 15331815]
- Snow R. W., Marsh K. The Consequences of Reducing Plasmodium falciparum Transmission in Africa. Advances in Parasitology. 2002;52:235–64. [PubMed: 12521262]
- Snow R. W., Menon A., Greenwood B. M. Measuring Morbidity from Malaria. Annals of Tropical Medicine and Parasitology. 1989;83:321–23. [PubMed: 2604470]
- Snow R. W., Trape J. F., Marsh K. The Past, Present, and Future of Childhood Malaria Mortality in Africa. Trends in Parasitology. 2001;17:593–97. [PubMed: 11756044]
- Steketee R. W., Nahlen B. L., Parise M. E., Menendez C. The Burden of Malaria in Pregnancy in Malaria-Endemic Areas. American Journal of Tropical Medicine and Hygiene. 2001;64(Suppl.):28–35. [PubMed: 11425175]
- Steketee R. W., Wirima J. J., Hightower A. W., Slutsker L., Heymann D. L., Breman J. G. The Effect of Malaria and Malaria Prevention in Pregnancy on Offspring Birthweight, Prematurity, and Intrauterine Growth Retardation in Rural Malawi. American Journal of Tropical Medicine and Hygiene. 1996;55(Suppl.):33–41. [PubMed: 8702035]
- Sudre P., Breman J. G., McFarland D., Koplan J. P. Treatment of Chloroquine Resistant Malaria in African Children: A Cost-Effectiveness Analysis. International Journal of Epidemiology. 1992;21:146–54. [PubMed: 1544746]
- Tang L. Progress in Malaria Control in China. Chinese Medical Journal. 2000;113(1):89–92. [PubMed: 11775219]
- Ter Kuile F. O., Parise M., Verhoef F., Udhayakumar V., Newman R. D., Van Eijk A. M. et al. The Burden of Co-infection with Human Immunodeficiency Virus Type 1 and Malaria in Pregnant Women in Sub-Saharan Africa. American Journal of Tropical Medicine and Hygiene. 2004;70(Suppl. 2):41–54. [PubMed: 15331818]
- Ter Kuile F. O., Terlouw D. J., Kariuki S. K., Phillips-Howard P. A., Mirel L. B., Hawley W. A. et al. Impact of Permethrin-Treated Bednets on Malaria, Anemia, and Growth in Infants in an Area of Intense Perennial Malaria Transmission in Western Kenya. American Journal of Tropical Medicine and Hygiene. 2003;68(Suppl. 4):68–77. [PubMed: 12749488]
- Thera M. A., Sehdev P. S., Coulibaly D., Traore K., Garba M. N. et al. Impact of Trimethoprim-Sulfamethoxa-Infectious Diseases. Journal of Infections Diseases. 2005;192(10):1823–29. [PMC free article: PMC2740817] [PubMed: 16235184]
- Warhurst, D. C., and J. E. Williams. 2004. "Laboratory Procedures for Diagnosis of Malaria." In Malaria: A Hematological Perspective, ed. S. H. Abdalla and G. Pasvol, 1–27, London: Imperial College Press.
- White N. J. Intermittent Presumptive Treatment for Malaria. PLoS Medicine. 2005;2(1):63. [PMC free article: PMC545196] [PubMed: 15696210]
- White, N. J., and J. G. Breman. 2005. "Malaria and Babesiosis: Diseases Caused by Red Blood Cell Parasites." In Harrison's Principles of Internal Medicine, 16th ed., ed. D. Kasper, E. Braunwald, A. S. Fauci, S. L. Hauser, D. L. Longo, and J. L. Jameson, 1218–33. McGraw-Hill: New York.
- Whitworth J., Morgan D., Quigley M., Smith A., Mayania B., Eotu H. et al. Effect of HIV-1 and Increasing Immunosuppression on Malaria Parasitaemia and Clinical Episodes in Adults in Rural Uganda: A Cohort Study. Lancet. 2000;356(9235):1051–56. [PubMed: 11009139]
- WHO (World Health Organization). 1996. Investing in Health Research and Development: Report of the Ad Hoc Committee on Health Research Relating to Future Intervention Options. TDR/Gen/96.1. Geneva: WHO.
- ———. 2000a. Twentieth Report of the WHO Expert Committee on Malaria. Technical Report Series 892. Geneva: WHO. [PubMed: 10892307]
- ———.2000bSevere and Complicated Malaria Transactions of the Royal Society of Tropical Medicine and Hygiene 95Suppl.51–90.
- ———. 2001a. Antimalarial Drug Combination Therapy. Report of a WHO Technical Consultation 4–5 April, 2001. WHO/CDS/RBM/2001.35. Geneva: WHO.
- ———. 2001b. "The Use of Antimalarial Drugs." Report of a WHO Informal Consultation, WHO, Geneva.
- ———. 2002a. Monitoring Antimalarial Drug Resistance. WHO/CDS/CSR/EPH/2002.1 WHO/CDS/RBM/2002.39. Geneva: WHO.
- ———. 2002b. World Health Report 2002: Reducing Risks, Promoting Healthy Life. Geneva: WHO. [PubMed: 14741909]
- ———. 2002c. South African Malaria Control Programme. Roll Back Malaria in Southern Africa. Baseline Survey 2001. Harare, Zimbabwe: WHO.
- ———. 2003a. Access to Antimalarial Medicines: Improving the Affordability and Financing of Artemisinin-Based Combination Therapies. Geneva: WHO.
- ———. 2003b. The Africa Malaria Report 2003. Geneva: WHO; New York: United Nations Children's Fund.
- ———. 2003c. The Scientific Working Group on Malaria Research. TWR/SWG/03.03. Geneva: WHO.
- ———. 2005. The World Malaria Report 2005: Roll Back Malaria. Geneva: WHO.
- World Bank. 2003. 2003 World Development Indicators. Washington, DC: World Bank.
- ———. 2005. Rolling Back Malaria, Global Strategy and Booster Program. Washington, DC: World Bank.
- Worrall E., Rietveld A., Delacollette C. The Burden of Malaria Epidemics and Cost-Effectiveness of Interventions in Epidemic Situations in Africa. American Journal of Tropical Medicine and Hygiene. 2004;71(Suppl. 2):136–40. [PubMed: 15331829]
- Yeung S., Pongtavornpinyo W., Hastings I. M., Mills A. J., White N. J. Antimalarial Drug Resistance, Artemisinin-Based Combination Therapy (ACT), and the Contribution of Modeling to Elucidating Policy Choices. American Journal of Tropical Medicine and Hygiene. 2004;71(Suppl. 2):179–86. [PubMed: 15331836]
- Review Conquering the intolerable burden of malaria: what's new, what's needed: a summary.[Am J Trop Med Hyg. 2004]Review Conquering the intolerable burden of malaria: what's new, what's needed: a summary.Breman JG, Alilio MS, Mills A. Am J Trop Med Hyg. 2004 Aug; 71(2 Suppl):1-15.
- Review Diagnosis and Treatment of the Febrile Child.[Reproductive, Maternal, Newbor...]Review Diagnosis and Treatment of the Febrile Child.Herlihy JM, D’Acremont V, Hay Burgess DC, Hamer DH. Reproductive, Maternal, Newborn, and Child Health: Disease Control Priorities, Third Edition (Volume 2). 2016 Apr 5
- Review Coverage of intermittent preventive treatment and insecticide-treated nets for the control of malaria during pregnancy in sub-Saharan Africa: a synthesis and meta-analysis of national survey data, 2009-11.[Lancet Infect Dis. 2013]Review Coverage of intermittent preventive treatment and insecticide-treated nets for the control of malaria during pregnancy in sub-Saharan Africa: a synthesis and meta-analysis of national survey data, 2009-11.van Eijk AM, Hill J, Larsen DA, Webster J, Steketee RW, Eisele TP, ter Kuile FO. Lancet Infect Dis. 2013 Dec; 13(12):1029-42. Epub 2013 Sep 18.
- [Current malaria situation in the Republic of Kazakhstan].[Med Parazitol (Mosk). 2001][Current malaria situation in the Republic of Kazakhstan].Bismil'din FB, Shapieva ZhZh, Anpilova EN. Med Parazitol (Mosk). 2001 Jan-Mar; (1):24-33.
- Use of intermittent preventive treatment for malaria in pregnancy in a rural area of western Kenya with high coverage of insecticide-treated bed nets.[Trop Med Int Health. 2005]Use of intermittent preventive treatment for malaria in pregnancy in a rural area of western Kenya with high coverage of insecticide-treated bed nets.van Eijk AM, Blokland IE, Slutsker L, Odhiambo F, Ayisi JG, Bles HM, Rosen DH, Adazu K, Lindblade KA. Trop Med Int Health. 2005 Nov; 10(11):1134-40.
- Conquering Malaria - Disease Control Priorities in Developing CountriesConquering Malaria - Disease Control Priorities in Developing Countries
Your browsing activity is empty.
Activity recording is turned off.
See more...