Summary
Clinical characteristics.
GAN-related neurodegeneration comprises a phenotypic continuum ranging from severe (sometimes called classic giant axonal neuropathy) to milder pure early-onset peripheral motor and sensory neuropathies. The classic giant axonal neuropathy phenotype typically manifests as an infantile-onset neurodegenerative disorder, starting as a severe peripheral motor and sensory neuropathy and evolving into central nervous system impairment (intellectual disability, seizures, cerebellar signs, and pyramidal tract signs). Most affected individuals become wheelchair dependent in the second decade of life and eventually bedridden with severe polyneuropathy, ataxia, and dementia. Death usually occurs in the third decade. At the milder end of the spectrum are predominantly motor and sensory neuropathies (with little to no CNS involvement) that overlap with the axonal form of Charcot-Marie-Tooth neuropathies.
Diagnosis/testing.
The diagnosis of GAN-related neuropathy is established in a proband with suggestive findings and biallelic GAN pathogenic variants identified by molecular genetic testing.
Management.
Treatment of manifestations: Supportive care is focused on managing the clinical findings of the individual, and often involves a team including neurologists, orthopedic surgeons, physiotherapists, occupational and physical therapists, psychologists, and speech-language pathologists. Major goals are to optimize intellectual and physical development.
Surveillance: Surveillance is individualized to monitor response to ongoing interventions and identify new manifestations.
Genetic counseling.
GAN-related neuropathy is inherited in an autosomal recessive manner. If both parents are known to be heterozygous for a GAN pathogenic variant, each sib of an affected individual has at conception a 25% chance of inheriting biallelic pathogenic variants and being affected, a 50% chance of being heterozygous, and a 25% chance of inheriting neither of the familial pathogenic variants. Once the GAN pathogenic variants have been identified in an affected family member, carrier testing for at-risk family members, prenatal testing for a pregnancy at increased risk, and preimplantation genetic testing are possible.
Diagnosis
No consensus clinical diagnostic criteria for GAN-related neurodegeneration have been published.
Suggestive Findings
GAN-related neurodegeneration should be suspected in individuals with the following clinical, electrophysiologic, and brain imaging findings, and family history.
Clinical findings
- Early-onset peripheral motor and sensory neuropathy (in all individuals)
- Variable findings typically observed in classic giant axonal neuropathy:
- Infantile- to early childhood-onset CNS involvement that may include developmental delay / intellectual disability, cerebellar signs (ataxia, oculomotor involvement, nystagmus, dysarthria), and/or pyramidal tract signs (i.e., spasticity)
- Kinky hair. Tightly curled lackluster hair that differs markedly from that of the parents. In individuals with milder phenotypes the hair tends to be mildly curly rather than frizzled or kinky [Bharucha-Goebel et al 2021].
Electrophysiologic findings
- Nerve conduction studies often show normal to moderately reduced nerve conduction velocity but severely reduced compound motor action potentials and absent sensory nerve action potentials.
- Auditory brain stem evoked responses, visual evoked responses, and somatosensory evoked responses are often abnormal
Brain imaging
- Classic giant axonal neuropathy. In early stages of classic GAN, brain MRI shows hyperintense signal in the cerebellar white matter surrounding the dentate nucleus [Bharucha-Goebel et al 2021]. As the condition advances, high signals on T2- weighted sequences in the anterior and posterior periventricular regions as well as in the cerebellar white matter are often seen [Demir et al 2005, Echaniz-Laguna et al 2020, Bharucha-Goebel et al 2021].Brain MRI and magnetic resonance spectroscopy (MRS) reveal evidence of significant demyelination and glial proliferation in the white matter, but no neuroaxonal loss [Brenner et al 2008, Ravishankar et al 2009].
- Intermediate phenotype. MRI shows mild and diffuse T2-weighted signal abnormalities in the posterior limb of the internal capsule and periventricular white matter. Infratentorial signal abnormalities are observed in the dentate nucleus and middle cerebellar peduncle [Abu-Rashid et al 2013].
- Milder phenotypes. Brain MRI findings vary from normal in most individuals to signs of mild cerebral and cerebellar atrophy [Zemmouri et al 2000, Aharoni et al 2016, Koichihara et al 2016, Bharucha-Goebel et al 2021].
Milder phenotypes of GAN-related neurodegeneration could be considered in individuals with early-onset peripheral motor and sensory neuropathy where work up for Charcot-Marie-Tooth disorders has proved inconclusive.
Family history is consistent with autosomal recessive inheritance (e.g., affected sibs and/or parental consanguinity). Absence of a known family history does not preclude the diagnosis.
Establishing the Diagnosis
The diagnosis of GAN-related neurodegeneration is established in a proband with suggestive findings and biallelic pathogenic (or likely pathogenic) variants in GAN identified by molecular genetic testing (see Table 1).
Note: (1) Per ACMG/AMP variant interpretation guidelines, the terms "pathogenic variants" and "likely pathogenic variants" are synonymous in a clinical setting, meaning that both are considered diagnostic and both can be used for clinical decision making [Richards et al 2015]. Reference to "pathogenic variants" in this section is understood to include any likely pathogenic variants. (2) Identification of biallelic GAN variants of uncertain significance (or identification of one known GAN pathogenic variant and one GAN variant of uncertain significance) does not establish or rule out a diagnosis of GAN-related neuropathy.
Molecular genetic testing approaches can include a combination of gene-targeted testing (single-gene testing or multigene panel) and comprehensive genomic testing (chromosomal microarray analysis, exome sequencing, genome sequencing) depending on the phenotype. Gene-targeted testing requires that the clinician determine which gene(s) are likely involved (Option 1), whereas genomic testing does not (Option 2).
Option 1
Single-gene testing. In individuals with suggestive findings of GAN-related neurodegeneration, sequence analysis of GAN is performed first to detect small intragenic deletions/insertions and missense, nonsense, and splice site variants. Note: Depending on the sequencing method used, single-exon, multiexon, or whole-gene deletions/duplications may not be detected. If only one or no variant is detected by the sequencing method used, the next step is to perform gene-targeted deletion/duplication analysis to detect exon and whole-gene deletions or duplications.
A neuropathy multigene panel that includes GAN and other genes of interest (see Differential Diagnosis) is most likely to identify the genetic cause of the condition while limiting identification of variants of uncertain significance and pathogenic variants in genes that do not explain the underlying phenotype. Note: (1) The genes included in the panel and the diagnostic sensitivity of the testing used for each gene vary by laboratory and are likely to change over time. (2) Some multigene panels may include genes not associated with the condition discussed in this GeneReview. (3) In some laboratories, panel options may include a custom laboratory-designed panel and/or custom phenotype-focused exome analysis that includes genes specified by the clinician. (4) Methods used in a panel may include sequence analysis, deletion/duplication analysis, and/or other non-sequencing-based tests.
For an introduction to multigene panels click here. More detailed information for clinicians ordering genetic tests can be found here.
Option 2
Comprehensive genomic testing does not require the clinician to determine which gene is likely involved. Exome sequencing is most commonly used; genome sequencing is also possible.
For an introduction to comprehensive genomic testing click here. More detailed information for clinicians ordering genomic testing can be found here.
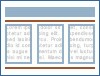
Table 1.
Molecular Genetic Testing Used in GAN-Related Neurodegeneration
Clinical Characteristics
Clinical Description
GAN-related neurodegeneration, caused by biallelic GAN pathogenic variants, encompasses a phenotypic continuum ranging from classic giant axonal neuropathy (GAN) – a neurodegenerative disorder affecting both the peripheral and central nervous systems at the severe end – to milder phenotypes with early-onset peripheral motor and sensory neuropathy with or without other findings. These phenotypes do not necessarily run true in families; in one family, phenotypes in family members with the same biallelic GAN pathogenic variants ranged from classic giant axonal neuropathy to milder peripheral neuropathy presentations [Tazir et al 2009].
To date, approximately 50 families have been identified with biallelic GAN pathogenic variants [Ben Hamida et al 1990, Zemmouri et al 2000, Kuhlenbäumer et al 2002, Abu-Rashid et al 2013, Koichihara et al 2016, Aharoni et al 2016, Hoebeke et al 2018, Didonna & Opal 2019, Echaniz-Laguna et al 2020, Bharucha-Goebel et al 2021].
Table 2 outlines the findings of classic giant axonal neuropathy, the first described phenotype, which is now known to be at the severe end of the GAN-related neurodegeneration phenotypic continuum.
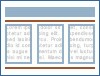
Table 2.
Select Features of Severe GAN-Related Neurodegeneration
Classic Giant Axonal Neuropathy
Neurologic findings. Classic giant axonal neuropathy, the phenotype first recognized in the GAN-related neurodegeneration spectrum, starts as severe peripheral motor and sensory neuropathy before age five years and evolves into central nervous system (CNS) impairment (intellectual disability, seizures, cerebellar signs, and pyramidal tract signs).
The motor and sensory peripheral neuropathy may also involve the cranial nerves, resulting in facial weakness, optic atrophy, and ophthalmoplegia. Tendon reflexes are often absent; Babinski sign may be present as a result of CNS involvement.
The majority of affected individuals show signs of CNS involvement including intellectual disability, cerebellar signs (ataxia, nystagmus, and dysarthria), epileptic seizures, and pyramidal tract signs (i.e., spasticity). Early intellectual development is nearly normal in many affected children, enabling them to attend a normal school initially; however, significant intellectual impairment usually occurs before the second decade of life.
Most affected individuals become wheelchair dependent in the second decade of life and eventually bedridden with severe polyneuropathy, ataxia, and dementia. In those with the most severe manifestations, death usually occurs in the third decade, typically the result of secondary complications such as respiratory failure.
Hair. Most individuals with classic giant axonal neuropathy have characteristic tightly curled lackluster hair that differs markedly from that of their parents. Microscopic examination of unstained hair shows abnormal variation in shaft diameter and twisting (pili torti) similar to the abnormality seen in Menkes disease; it can also show longitudinal grooves on scanning electron microscopy [Kennerson et al 2010, Kaler 2011, Yi et al 2012].
Milder GAN-Related Neurodegeneration Phenotypes
Milder phenotypes have been reported with later age of onset, extended survival, or modest CNS deterioration with pathology reminiscent of Charcot-Marie-Tooth axonal disorders, sometimes referred to as axonal CMT (plus) syndrome [Ben Hamida et al 1990, Zemmouri et al 2000, Aharoni et al 2016, Hoebeke et al 2018, Bharucha-Goebel et al 2021].
Genotype-Phenotype Correlations
No genotype-phenotype correlations have been reported for GAN-related neurodegeneration. Famiy members with the same pathogenic variant who had different clinical phenotypes have been reported [Tazir et al 2009].
Nomenclature
The term "axonal CMT (plus) syndrome" may be used to refer to predominantly motor and sensory neuropathy phenotypes (with little to no CNS involvement) that overlap with axonal forms of Charcot-Marie-Tooth neuropathy [Bharucha-Goebel et al 2021].
Prevalence
To date, about 50 families with GAN-related neurodegeneration have been reported worldwide. The actual prevalence is not known.
Genetically Related (Allelic) Disorders
No phenotypes other than those discussed in this GeneReview are known to be associated with germline pathogenic variants in GAN.
Differential Diagnosis
Hereditary Disorders in the Differential Diagnosis of Classic Giant Axonal Neuropathy
At onset, classic giant axonal neuropathy (GAN) presents with features (e.g., neuropathic deficits, giant axons, and neurofilament aggregation) similar to those associated with several severe, early-onset hereditary motor and sensory neuropathies [Lescouzères & Bomont 2020]. These neuropathies and other disorders of interest in the differential diagnosis of classic GAN are summarized in Table 3.
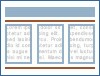
Table 3.
Genes of Interest in the Differential Diagnosis of Classic Giant Axonal Neuropathy
Differential Diagnosis of Milder GAN-Related Neurodegeneration Phenotypes
Milder GAN-related neurodegeneration phenotypes overlap with Charcot-Marie-Tooth (CMT) hereditary neuropathy and should be considered in the broader differential diagnosis of CMT. More than 80 different genes are associated with CMT (see Causes of Charcot-Marie-Tooth Hereditary Neuropathy) with a subset of these associated with giant axons, even more closely mimicking GAN-related neurodegeneration [Fabrizi et al 2004, Azzedine et al 2006, Ylikallio et al 2013, Klein et al 2014].
Management
No clinical practice guidelines for GAN-related neurodegeneration have been published.
Evaluations Following Initial Diagnosis
To establish the extent of disease and needs in an individual diagnosed with GAN-related neurodegeneration, the evaluations summarized in Table 4 (if not performed as part of the evaluation that led to the diagnosis) are recommended.
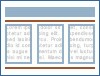
Table 4.
Recommended Evaluations Following Initial Diagnosis in Individuals with GAN-Related Neurodegeneration
Treatment of Manifestations
Supportive care is focused on managing the clinical findings of the individual, and often involves a team including (pediatric) neurologists, orthopedic surgeons, physiotherapists, occupational and physical therapists, psychologists, and speech-language pathologists. Major goals are to optimize intellectual and physical development.
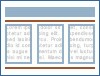
Table 5.
Treatment of Manifestations in Individuals with GAN-Related Neurodegeneration
Developmental Delay / Intellectual Disability Management Issues
The following information represents typical management recommendations for individuals with developmental delay / intellectual disability in the United States; standard recommendations may vary from country to country.
Ages 0-3 years. Referral to an early intervention program is recommended for access to occupational, physical, speech, and feeding therapy as well as infant mental health services, special educators, and sensory impairment specialists. In the US, early intervention is a federally funded program available in all states that provides in-home services to target individual therapy needs.
Ages 3-5 years. In the US, developmental preschool through the local public school district is recommended. Before placement, an evaluation is made to determine needed services and therapies and an individualized education plan (IEP) is developed for those who qualify based on established motor, language, social, or cognitive delay. The early intervention program typically assists with this transition. Developmental preschool is center based; for children too medically unstable to attend, home-based services are provided.
All ages. Consultation with a developmental pediatrician is recommended to ensure the involvement of appropriate community, state, and educational agencies (US) and to support parents in maximizing quality of life. Some issues to consider:
- IEP services:
- An IEP provides specially designed instruction and related services to children who qualify.
- IEP services will be reviewed annually to determine whether any changes are needed.
- Special education law requires that children participating in an IEP be in the least restrictive environment feasible at school and included in general education as much as possible, when and where appropriate.
- When relevant, vision and hearing consultants should be a part of the child's IEP team to support access to academic material.
- PT, OT, and speech services will be provided in the IEP to the extent that the need affects the child's access to academic material. Beyond that, private supportive therapies based on the affected individual's needs may be considered. Specific recommendations regarding type of therapy can be made by a developmental pediatrician.
- As a child enters the teen years, a transition plan should be discussed and incorporated in the IEP. For those receiving IEP services, the public school district is required to provide services until age 21.
- A 504 plan (Section 504: a US federal statute that prohibits discrimination based on disability) can be considered for those who require accommodations or modifications such as front-of-class seating, assistive technology devices, classroom scribes, extra time between classes, modified assignments, and enlarged text.
- Developmental Disabilities Administration (DDA) enrollment is recommended. DDA is a US public agency that provides services and support to qualified individuals. Eligibility differs by state but is typically determined by diagnosis and/or associated cognitive/adaptive disabilities.
- Families with limited income and resources may also qualify for supplemental security income (SSI) for their child with a disability.
Communication issues. Consider evaluation for alternative means of communication (e.g., augmentative and alternative communication [AAC]) for individuals who have expressive language difficulties. An AAC evaluation can be completed by a speech-language pathologist who has expertise in the area. The evaluation will consider cognitive abilities and sensory impairments to determine the most appropriate form of communication. AAC devices can range from low-tech, such as picture exchange communication, to high-tech, such as voice-generating devices. Contrary to popular belief, AAC devices do not hinder verbal development of speech, but rather support optimal speech and language development.
Surveillance
Surveillance is individualized and involves monitoring response to ongoing interventions and identifying new manifestations. Surveillance may involve some or all of the recommendations in Table 6.
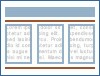
Table 6.
Recommended Surveillance at Each Visit for Individuals with GAN-Related Neurodegeneration
Evaluation of Relatives at Risk
See Genetic Counseling for issues related to testing of at-risk relatives for genetic counseling purposes.
Therapies Under Investigation
Search ClinicalTrials.gov in the US and EU Clinical Trials Register in Europe for access to information on clinical studies for a wide range of diseases and conditions. Note: There may not be clinical trials for this disorder.
Genetic Counseling
Genetic counseling is the process of providing individuals and families with information on the nature, mode(s) of inheritance, and implications of genetic disorders to help them make informed medical and personal decisions. The following section deals with genetic risk assessment and the use of family history and genetic testing to clarify genetic status for family members; it is not meant to address all personal, cultural, or ethical issues that may arise or to substitute for consultation with a genetics professional. —ED.
Mode of Inheritance
GAN-related neurodegeneration is inherited in an autosomal recessive manner.
Risk to Family Members
Parents of a proband
- The parents of an affected individual are typically heterozygotes (i.e., carriers of one GAN pathogenic variant).
- Molecular genetic testing is recommended for the parents of a proband to confirm that both parents are heterozygous for a GAN pathogenic variant and to allow reliable recurrence risk assessment. If a pathogenic variant is detected in only one parent:
- And the child has compound heterozygous GAN pathogenic variants, the child may have one inherited variant and one de novo pathogenic variant [Shi et al 2020].
- And the child appears to have homozygous GAN pathogenic variants, possible explanations include a large deletion on one allele (if not previously tested for) and uniparental isodisomy for chromosome 16 [Miyatake et al 2015].
- Heterozygotes are clinically asymptomatic but can display mild axonal neuropathy, as revealed by moderate reduction of nerve action potential amplitudes [Demir et al 2005].
Sibs of a proband
- If both parents are known to be heterozygous for a GAN pathogenic variant, each sib of an affected individual has at conception a 25% chance of inheriting biallelic pathogenic variants and being affected, a 50% chance of being heterozygous, and a 25% chance of inheriting neither of the familial pathogenic variants.
- Significant intrafamilial clinical variability has been reported in GAN-related neurodegeneration. In one family, phenotypes in family members with the same biallelic GAN pathogenic variants ranged from classic giant axonal neuropathy to milder peripheral neuropathy presentations [Tazir et al 2009].
- If the proband has GAN-related neurodegeneration as the result of uniparental isodisomy, only one parent is heterozygous for a GAN pathogenic variant, and neither parent has a chromosome rearrangement, each sib of an affected individual has at conception a 50% chance of being a clinically asymptomatic carrier and an approximately 50% chance of being unaffected and not a carrier; the risk to sibs of a proband of being affected is unknown but is presumed to be less than 1%.
Offspring of a proband
- Individuals with classic giant axonal neuropathy are not known to reproduce.
- The offspring of an individual with a milder GAN-related neurodegeneration phenotype are obligate heterozygotes (carriers) for a pathogenic variant in GAN.
Other family members. The risk to other family members depends on the status of the proband's parents: if a parent is heterozygous for a GAN pathogenic variant, the parent's family members are at risk of being carriers.
Carrier Detection
Carrier testing for at-risk relatives requires prior identification of the GAN pathogenic variants in the family.
Note: Some clinically asymptomatic heterozygotes can show mild axonal neuropathy, as revealed by moderate reduction of nerve action potential amplitudes [Demir et al 2005].
Related Genetic Counseling Issues
Family planning
- The optimal time for determination of genetic risk and discussion of the availability of prenatal/preimplantation genetic testing is before pregnancy.
- It is appropriate to offer genetic counseling (including discussion of potential risks to offspring and reproductive options) to young adults who are affected, are carriers, or are at risk of being carriers.
Prenatal Testing and Preimplantation Genetic Testing
Once the GAN pathogenic variants have been identified in an affected family member, prenatal testing for a pregnancy at increased risk and preimplantation genetic testing are possible.
Differences in perspective may exist among medical professionals and within families regarding the use of prenatal testing. While most centers would consider use of prenatal testing to be a personal decision, discussion of these issues may be helpful.
Resources
GeneReviews staff has selected the following disease-specific and/or umbrella support organizations and/or registries for the benefit of individuals with this disorder and their families. GeneReviews is not responsible for the information provided by other organizations. For information on selection criteria, click here.
- Hannah's Hope Fund for Giant Axonal Neuropathy19 Blue Jay WayRexford NY 12148Phone: 518-383-9053Email: lorisames@yahoo.com
- MedlinePlus
- Association CMT FranceFrancePhone: 820 077 540; 2 47 27 96 41
- Charcot-Marie-Tooth Association (CMTA)Phone: 800-606-2682Email: info@cmtausa.org
- Child Neurology FoundationPhone: 888-417-3435Email: programs@childneurologyfoundation.org
- European Neuromuscular Centre (ENMC)NetherlandsPhone: 31 35 5480481Email: enmc@enmc.org
- Hereditary Neuropathy FoundationPhone: 855-435-7268 (toll-free); 212-722-8396Fax: 917-591-2758Email: info@hnf-cure.org
Molecular Genetics
Information in the Molecular Genetics and OMIM tables may differ from that elsewhere in the GeneReview: tables may contain more recent information. —ED.
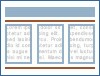
Table A.
GAN-Related Neurodegeneration: Genes and Databases
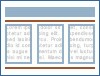
Table B.
OMIM Entries for GAN-Related Neurodegeneration (View All in OMIM)
Molecular Pathogenesis
GAN encodes gigaxonin, a BTB-Kelch protein [Adams et al 2000]. Gigaxonin controls the turnover of specific proteins by targeting them to the proteasome for degradation. While all the substrates have yet to be identified, a key substrate, cytoskeletal intermediate filaments, which include neurofilaments (NFs), have been found to accumulate in neurons and glial fibrillary acidic protein (GFAP) in glial cells [Opal & Goldman 2013, Israeli et al 2016, Didonna & Opal 2019]. Accumulation of these proteins impairs mitochondrial bioenergetics, organelle motility, and cell survival [Israeli et al 2016]. Although gigaxonin also interacts with three proteins involved in microtubule (MT) homeostasis and dynamics (the MT-associated proteins MAP1B and MAP1S, and the tubulin chaperone TBCB) [Ding et al 2002, Allen et al 2005, Wang et al 2005, Ding et al 2006, Cleveland et al 2009], studies suggest that they do not play an important role in GAN-related neurodegeneration. More recent evidence suggests that GAN controls the levels of Atg16L involved in autophagy [Scrivo et al 2019].
Mechanism of disease causation. Loss of function
Chapter Notes
Author Notes
An overview of current work in the lab and contact information can be found on our website.
Acknowledgments
We acknowledge the generous funding from the Hannah’s Hope Fund, the Muscular Dystrophy Association, and the NIH, which supports our ongoing research and understanding of GAN-related neurodegeneration and other neurologic disorders.
Author History
Pascale Bomont, PhD; Institute for Neurosciences of Montpellier (2012-2021)
Gregor Kuhlenbäumer, MD, PhD; University of Kiel (2003-2021)
Puneet Opal, MD, PhD (2021-present)
Vincent Timmerman, PhD; University of Antwerp (2003-2021)
Revision History
- 14 October 2021 (bp) Comprehensive update posted live
- 9 October 2014 (me) Comprehensive update posted live
- 21 June 2012 (me) Comprehensive update posted live
- 11 August 2009 (cd) Revision: sequence analysis available clinically
- 2 July 2007 (me) Comprehensive update posted live
- 28 February 2005 (me) Comprehensive update posted live
- 9 January 2003 (me) Review posted live
- 5 August 2002 (vt) Original submission
References
Literature Cited
- Abu-Rashid M, Mahajnah M, Jaber L, Kornreich L, Bar-On E, Basel-Vanagaite L, Soffer D, Koenig M, Straussberg R. A novel mutation in the GAN gene causes an intermediate form of giant axonal neuropathy in an Arab-Israeli family. Eur J Paediatr Neurol. 2013;17:259–64. [PubMed: 23332420]
- Adams J, Kelso R, Cooley L. The kelch repeat superfamily of proteins: propellers of cell function. Trends Cell Biol. 2000;10:17–24. [PubMed: 10603472]
- Aharoni S, Barwick KE, Straussberg R, Harlalka GV, Nevo Y, Chioza BA, McEntagart MM, Mimouni-Bloch A, Weedon M, Crosby AH. Novel homozygous missense mutation in GAN associated with Charcot-Marie-Tooth disease type 2 in a large consanguineous family from Israel. BMC Med Genet. 2016;17:82. [PMC free article: PMC5112725] [PubMed: 27852232]
- Allen E, Ding J, Wang W, Pramanik S, Chou J, Yau V, Yang Y. Gigaxonin-controlled degradation of MAP1B light chain is critical to neuronal survival. Nature. 2005;438:224–8. [PubMed: 16227972]
- Azzedine H, Ravisé N, Verny C, Gabrëels-Festen A, Lammens M, Grid D, Vallat JM, Durosier G, Senderek J, Nouioua S, Hamadouche T, Bouhouche A, Guilbot A, Stendel C, Ruberg M, Brice A, Birouk N, Dubourg O, Tazir M, LeGuern E. Spine deformities in Charcot-Marie-Tooth 4C caused by SH3TC2 gene mutations. Neurology. 2006;67:602–6. [PubMed: 16924012]
- Ben Hamida M, Hentati F, Ben Hamida C. Giant axonal neuropathy with inherited multisystem degeneration in a Tunisian kindred. Neurology. 1990;40:245–50. [PubMed: 2153943]
- Bharucha-Goebel DX, Norato G, Saade D, Paredes E, Biancavilla V, Donkervoort S, Kaur R, Lehky T, Fink M, Armao D, Gray SJ, Waite M, Debs S, Averion G, Hu Y, Zein WM, Foley AR, Jain M, Bönnemann CG. Giant axonal neuropathy: cross sectional analysis of a large natural history cohort. Brain. 2021;144:3239–50. [PMC free article: PMC8634068] [PubMed: 34114613]
- Brenner C, Speck-Martins CE, Farage L, Barker PB. 3T MR with diffusion tensor imaging and single-voxel spectroscopy in giant axonal neuropathy. J Magn Reson Imaging. 2008;28:236–41. [PMC free article: PMC2947443] [PubMed: 18581347]
- Bürk K, Sival DA. Scales for the clinical evaluation of cerebellar disorders. Handb Clin Neurol. 2018;154:329–39. [PubMed: 29903450]
- Cleveland DW, Yamanaka K, Bomont P. Gigaxonin controls vimentin organization through a tubulin chaperone-independent pathway. Hum Mol Genet. 2009;18:1384–94. [PMC free article: PMC2664145] [PubMed: 19168853]
- Demir E, Bomont P, Erdem S, Cavalier L, Demirci M, Kose G, Muftuoglu S, Cakar AN, Tan E, Aysun S, Topcu M, Guicheney P, Koenig M, Topaloglu H. Giant axonal neuropathy: clinical and genetic study in six cases. J Neurol Neurosurg Psychiatry. 2005;76:825–32. [PMC free article: PMC1739689] [PubMed: 15897506]
- Didonna A, Opal P. The role of neurofilament aggregation in neurodegeneration: lessons from rare inherited neurological disorders. Mol Neurodegener. 2019;14:19. [PMC free article: PMC6524292] [PubMed: 31097008]
- Ding J, Allen E, Wang W, Valle A, Wu C, Nardine T, Cui B, Yi J, Taylor A, Jeon NL, Chu S, So Y, Vogel H, Tolwani R, Mobley W, Yang Y. Gene targeting of GAN in mouse causes a toxic accumulation of microtubule-associated protein 8 and impaired retrograde axonal transport. Hum Mol Genet. 2006;15:1451–63. [PubMed: 16565160]
- Ding J, Liu JJ, Kowal AS, Nardine T, Bhattacharya P, Lee A, Yang Y. Microtubule-associated protein 1B: a neuronal binding partner for gigaxonin. J Cell Biol. 2002;158:427–33. [PMC free article: PMC2173828] [PubMed: 12147674]
- Echaniz-Laguna A, Cuisset JM, Guyant-Marechal L, Aubourg P, Kremer L, Baaloul N, Verloes A, Beladgham K, Perrot J, Francou B, Latour P. Giant axonal neuropathy: a multicenter retrospective study with genotypic spectrum expansion. Neurogenetics. 2020;21:29–37. [PubMed: 31655922]
- Fabrizi GM, Cavallaro T, Angiari C, Bertolasi L, Cabrini I, Ferrarini M, Rizzuto N. Giant axon and neurofilament accumulation in Charcot-Marie-Tooth disease type 2E. Neurology. 2004;62:1429–31. [PubMed: 15111691]
- Hoebeke C, Bonello-Palot N, Audic F, Boulay C, Tufod D, Attarian S, Chabrol B. Retrospective study of 75 children with peripheral inherited neuropathy: Genotype-phenotype correlations. Arch Pediatr. 2018;25:452–8. [PubMed: 30340945]
- Israeli E, Dryanovski DI, Schumacker PT, Chandel NS, Singer JD, Julien JP, Goldman RD, Opal P. Intermediate filament aggregates cause mitochondrial dysmotility and increase energy demands in giant axonal neuropathy. Hum Mol Genet. 2016;25:2143–57. [PMC free article: PMC5081048] [PubMed: 27000625]
- Kaler SG. ATP7A-related copper transport diseases-emerging concepts and future trends. Nat Rev Neurol. 2011;7:15–29. [PMC free article: PMC4214867] [PubMed: 21221114]
- Kennerson ML, Nicholson GA, Kaler SG, Kowalski B, Mercer JF, Tang J, Llanos RM, Chu S, Takata RI, Speck-Martins CE, Baets J, Almeida-Souza L, Fischer D, Timmerman V, Taylor PE, Scherer SS, Ferguson TA, Bird TD, De Jonghe P, Feely SM, Shy ME, Garbern JY. Missense mutations in the copper transporter gene ATP7A cause X-linked distal hereditary motor neuropathy. Am J Hum Genet. 2010;86:343–52. [PMC free article: PMC2833394] [PubMed: 20170900]
- Klein CJ, Wu Y, Vogel P, Goebel HH, Bönnemann C, Zukosky K, Botuyan MV, Duan X, Middha S, Atkinson EJ, Mer G, Dyck PJ. Ubiquitin ligase defect by DCAF8 mutation causes HMSN2 with giant axons. Neurology. 2014;82:873–8. [PMC free article: PMC3959756] [PubMed: 24500646]
- Koichihara R, Saito T, Ishiyama A, Komaki H, Yuasa S, Saito Y, Nakagawa E, Sugai K, Shiihara T, Shioya A, Saito Y, Higuchi Y, Hashiguchi A, Takashima H, Sasaki M. A mild case of giant axonal neuropathy without central nervous system manifestation. Brain Dev. 2016;38:350–3. [PubMed: 26381321]
- Kuhlenbäumer G, Young P, Oberwittler C, Hünermund G, Schirmacher A, Domschke K, Ringelstein B, Stögbauer F. Giant axonal neuropathy (GAN): case report and two novel mutations in the gigaxonin gene. Neurology. 2002;58:1273–6. [PubMed: 11971098]
- Lescouzères L, Bomont P. E3 ubiquitin ligases in neurological diseases: focus on gigaxonin and autophagy. Front Physiol. 2020;11:1022. [PMC free article: PMC7642974] [PubMed: 33192535]
- Miyatake S, Tada H, Moriya S, Takanashi J, Hirano Y, Hayashi M, Oya Y, Nakashima M, Tsurusaki Y, Miyake N, Matsumoto N, Saitsu H. Atypical giant axonal neuropathy arising from a homozygous mutation by uniparental isodisomy. Clin Genet. 2015;87:395–7. [PubMed: 25040701]
- Opal P, Goldman RD. Explaining intermediate filament accumulation in giant axonal neuropathy. Rare Dis. 2013;1:e25378. [PMC free article: PMC3927486] [PubMed: 25003002]
- Ravishankar S, Goel G, Rautenstrauss CB, Nalini A. Spectrum of magnetic resonance imaging findings in a family with giant axonal neuropathy confirmed by genetic studies. Neurol India. 2009;57:181–4. [PubMed: 19439850]
- Richards S, Aziz N, Bale S, Bick D, Das S, Gastier-Foster J, Grody WW, Hegde M, Lyon E, Spector E, Voelkerding K, Rehm HL, et al. Standards and guidelines for the interpretation of sequence variants: a joint consensus recommendation of the American College of Medical Genetics and Genomics and the Association for Molecular Pathology. Genet Med. 2015;17:405–24. [PMC free article: PMC4544753] [PubMed: 25741868]
- Scrivo A, Codogno P, Bomont P. Gigaxonin E3 ligase governs ATG16L1 turnover to control autophagosome production. Nat Commun. 2019;10:780. [PMC free article: PMC6377711] [PubMed: 30770803]
- Shi M, Chen X, Zeng L, Li Z, Liang D, Wu L. The rare Alus element-mediated chimerism of multiple de novo complex rearrangement sequences in GAN result in giant axonal neuropathy. Clin Chim Acta. 2020;502:91–8. [PubMed: 31877298]
- Stenson PD, Mort M, Ball EV, Chapman M, Evans K, Azevedo L, Hayden M, Heywood S, Millar DS, Phillips AD, Cooper DN. The Human Gene Mutation Database (HGMD®): optimizing its use in a clinical diagnostic or research setting. Hum Genet. 2020;139:1197–207. [PMC free article: PMC7497289] [PubMed: 32596782]
- Tazir M, Nouioua S, Magy L, Huehne K, Assami S, Urtizberea A, Grid D, Hamadouche T, Rautenstrauss B, Vallat JM. Phenotypic variability in giant axonal neuropathy. Neuromuscul Disord. 2009;19:270–4. [PubMed: 19231187]
- Wang W, Ding J, Allen E, Zhu P, Zhang L, Vogel H, Yang Y. Gigaxonin interacts with tubulin folding cofactor B and controls its degradation through the ubiquitin-proteasome pathway. Curr Biol. 2005;15:2050–5. [PubMed: 16303566]
- Yi L, Donsante A, Kennerson ML, Mercer JF, Garbern JY, Kaler SG. Altered intracellular localization and valosin-containing protein (p97 VCP) interaction underlie ATP7A-related distal motor neuropathy. Hum Mol Genet. 2012;21:1794–807. [PMC free article: PMC3313796] [PubMed: 22210628]
- Ylikallio E, Pöyhönen R, Zimon M, De Vriendt E, Hilander T, Paetau A, Jordanova A, Lönnqvist T, Tyynismaa H. Deficiency of the E3 ubiquitin ligase TRIM2 in early-onset axonal neuropathy. Hum Mol Genet. 2013;22:2975–83. [PubMed: 23562820]
- Zemmouri R, Azzedine H, Assami S, Kitouni N, Vallat JM, Maisonobe T, Hamadouche T, Kessaci M, Mansouri B, Le Guern E, Grid D, Tazir M. Charcot-Marie-Tooth 2-like presentation of an Algerian family with giant axonal neuropathy. Neuromuscul Disord. 2000;10:592–8. [PubMed: 11053687]
Publication Details
Author Information and Affiliations
Publication History
Initial Posting: January 9, 2003; Last Update: October 14, 2021.
Copyright
GeneReviews® chapters are owned by the University of Washington. Permission is hereby granted to reproduce, distribute, and translate copies of content materials for noncommercial research purposes only, provided that (i) credit for source (http://www.genereviews.org/) and copyright (© 1993-2024 University of Washington) are included with each copy; (ii) a link to the original material is provided whenever the material is published elsewhere on the Web; and (iii) reproducers, distributors, and/or translators comply with the GeneReviews® Copyright Notice and Usage Disclaimer. No further modifications are allowed. For clarity, excerpts of GeneReviews chapters for use in lab reports and clinic notes are a permitted use.
For more information, see the GeneReviews® Copyright Notice and Usage Disclaimer.
For questions regarding permissions or whether a specified use is allowed, contact: ude.wu@tssamda.
Publisher
University of Washington, Seattle, Seattle (WA)
NLM Citation
Opal P. GAN-Related Neurodegeneration. 2003 Jan 9 [Updated 2021 Oct 14]. In: Adam MP, Feldman J, Mirzaa GM, et al., editors. GeneReviews® [Internet]. Seattle (WA): University of Washington, Seattle; 1993-2024.