The content of this book is licensed under a Creative Commons Attribution-NonCommercial-NoDerivs 4.0 Unported license. To view the terms and conditions of this license, visit https://creativecommons.org/licenses/by-nc-nd/4.0/
NCBI Bookshelf. A service of the National Library of Medicine, National Institutes of Health.
Varki A, Cummings RD, Esko JD, et al., editors. Essentials of Glycobiology [Internet]. 4th edition. Cold Spring Harbor (NY): Cold Spring Harbor Laboratory Press; 2022. doi: 10.1101/glycobiology.4e.31
The R-type lectin superfamily is characterized by a carbohydrate-recognition domain (CRD) originally reported in a polypeptide of ricin, a plant toxin. Ricin was the first lectin discovered, and the R-type lectins are named after it. Through evolution, the R-type lectin domain has given rise to various protein forms, some containing this domain alone and others containing additional functional domains (e.g., enzyme or toxin). We will discuss the diversity of this superfamily in terms of molecular tinkering and “bricolage,” based on structure–function relationships.
HISTORICAL BACKGROUND
In 1888, Peter Hermann Stillmark at the University of Dorpat (now University of Tartu, Estonia) reported that protein extracts from seeds of Ricinus communis (commonly called “castor oil plant”; seeds are called “castor beans”) contained a factor (which he termed “ricin”) capable of agglutinating erythrocytes. Ricin was already well known as a toxin, but its carbohydrate-binding specificity was not analyzed in detail until the mid-20th century, when it was classified as a lectin. Structural analysis of ricin, performed around a century after its discovery, showed that the CRD had a “β-trefoil” (three-leaved) fold with a common Q-x-W motif in each subdomain. This domain structure defines the R(icin)-type lectins, a superfamily also classified as carbohydrate-binding molecule (CBM) 13 (http://www.cazy.org/CBM13.html) in the Carbohydrate-Active Enzymes (CAZy) database (Chapter 6). These lectins are widely present in all three biological domains (and their viruses) as glycan-binding proteins, including enzymes and toxins (Figure 31.1).

FIGURE 31.1.
The R-type lectin superfamily. Different groups within the superfamily are characterized by the domain structures shown.
R-TYPE LECTINS IN PLANTS
General Properties of Ricin
Ricinus communis is native to Africa and India and has been used for millennia, by many cultural groups, for numerous medical and industrial purposes. Two lectins, originally termed RCA-I and RCA-II, can be purified from the seeds. RCA-I (molecular mass ∼120 kDa) is a hemagglutinin and a very weak toxin. RCA-II (∼60 kDa), better known as ricin, is an agglutinin and a very potent toxin. Ricin is easily extractable from castor beans and can kill humans at very small doses.
RCA-II (ricin; Figure 31.2) is synthesized as a single prepropolypeptide of 576 amino acid (aa) residues containing a secretion signal peptide (residues 1–35), an A chain (residues 36–302), a 12-aa linker region (residues 303–314), and a B chain (residues 315–576). The A-chain toxin is an N-glycoside hydrolase (EC 3.2.2.22) that inactivates the 60S ribosome. The B-chain lectin domain binds to galactose (Gal) and β-galactosides. The amino-terminal signal sequence brings the propolypeptide to the endoplasmic reticulum (ER), where the signal is cleaved off, and the polypeptide is glycosylated. The A chain is linked to the B chain by a disulfide bond between cysteine residues 294 and 318 (Cys4 in the free B chain), and four intrachain disulfide bonds are formed. After glycosylation, ricin is transported to protein storage bodies in castor beans, where the mature ricin protein is generated by removal of the linker peptide by an endopeptidase.

FIGURE 31.2.
Structure of ricin. (A) Ricinus communis (beans and plants). (B) Crystal structure of ricin (RCA-II); resolution 2.5 Å. (Rutenber E, et al. 1991. Proteins 10: 240–250. Image from PDB entry 2AAI.)
RCA-I (agglutinin) is a tetramer consisting of two noncovalently associated ricin-like heterodimers. Each heterodimer contains an A chain disulfide-linked to a Gal-binding B chain. The A-chain sequences of RCA-I and RCA-II differ in 18 residues and are 93% similar, whereas the B-chain sequences differ in 41 of 262 residues and are 84% similar. The subunits are all N-glycosylated and usually display oligomannose-type N-glycans. Several other lectins having high homology with ricin are also encoded by the R. communis genome; some of these are termed ricin-A, -B, -C, -D (ricin), and -E.
Carbohydrate-Binding Specificity of Ricin
The B chain of both RCA-I (agglutinin) and RCA-II (ricin) contains two CRDs in the polypeptide. RCA-I binds preferentially to terminal β-linked Gal, whereas RCA-II binds to either terminal β-linked Gal or GalNAc. These lectins are often purified and separated by differential elution from Gal-based affinity resins; RCA-II is eluted with GalNAc, and RCA-I is then eluted with Gal. These lectins have very low binding affinities for monosaccharides (Kd in the range 10−3 to 10−4 m), but very high affinities (10−7 to 10−8 m) for cells, because of their multivalency and enhanced binding to multiple surface glycans having nonreducing terminal Galβ1-4GlcNAc-R (type-2 LacNAc) sequences. Both lectins also bind strongly to glycans with GalNAcβ1-4GlcNAc-R (LacdiNAc), and weakly to the isomer Galβ1-3GlcNAc-R (type-1 LacNAc). Neither lectin binds appreciably to glycoconjugates having nonreducing terminal α-linked Gal residues.
Toxicity of Ricin
Ricin is highly toxic and the effects of ingestion are severe, with symptoms appearing after a 2- to 24-h latent period. The lethal dose (LD50) of ricin is extremely low (3–5 µg/kg body weight). Ricin is classified as a type II ribosome-inactivating protein (RIP-II). RCA-I (agglutinin) is less toxic than ricin, because of the weaker enzymatic activity of its A-chain. Type I ribosome-inactivating proteins (RIP-I), which lack a B chain with R-type lectin domains, are much less toxic than ricin because toxin entry into target cells is facilitated by B-chain carbohydrate-binding activity. RIP-I expressed in tissues of various types of plants affects disease resistance. Horizontal transmission of RIP-I gene to the genome of whitefly (Hemiptera, Insecta) feeding on such plants was recently demonstrated.
Ricin bound to a β-linked cell-surface Gal/GalNAc containing glycans is transported into endosomes (Figure 31.3), and then migrates by retrograde trafficking to ER via the trans-Golgi network and Golgi apparatus. In the ER, A and B chains separate following reduction of the disulfide bond, perhaps catalyzed by the enzyme protein disulfide isomerase. A portion of the free A chain, which may be partially denatured in the ER, escapes by retrotranslocation through the Sec61 translocon and enters the cytoplasm.
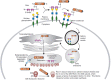
FIGURE 31.3.
Pathway of ricin uptake by cells and mechanism whereby toxic activity of A chain in cytoplasm results in cell death.
Just a few molecules of ricin A-chain in the cytoplasm are sufficient to kill cells. The catalytic subunit of A-chain is an rRNA N-glycosylase having eight α-helices and eight β-strands, which cleaves one purine base (adenine 4324) in the exposed GAGA tetraloop of the 28S RNA of the eukaryotic 60S ribosomal subunit. This deletion results in loss of binding of elongation factor-2, inability of ribosomes to perform protein synthesis, and consequent cell death.
Sequence of R-Type Lectin Domain
Sequence analysis of RCA-I and RCA-II revealed that their B-chains contain two similar, characteristic regions in the polypeptide (i.e., the tandem-repeat R-type lectin). Each of the domains is approximately 120 aa long, with three tandem repeats of a 40-residue subdomain. Each subdomain contains a Q-x-W motif (x represents any aa). This tandem repeat is present in other toxins from plants of various phyla and is evidently characteristic of plant lectins. In the late 1990s, similar sequences were discovered in lectins from nonplant organisms, indicating that the ricin-B-like pattern is universal. In the early 2000s, these lectins were classified as the “R-type lectin superfamily,” and this superfamily is now categorized as a single domain (Pfam: PF14200) or tandem-repeat domain (Pfam: PF00652).
β-Trefoil Fold
Crystal structure analysis of ricin (Figure 31.2) indicated that B-chain (PDB 2AAI) has a barbell-like shape with the two tandem R-type CRDs at the ends, ∼35 Å apart. R-type lectins have a fold termed “β-trefoil fold” (from the Latin “trifolium” meaning “three-leaved,” as seen for example in clover) (Figure 31.4). The β-trefoil fold presumably arose through triplication of an ancestral gene encoding the 40-residue subdomain. The three subdomains, termed α, β, and γ, each consist of 12 β-strands and loops arranged around a central axis, giving the protein internal pseudorotation symmetry. β-trefoil fold is maintained by the characteristic Q-x-W motif in each subdomain of ricin B-chain and common R-type lectins. More than 10% of lectins use this fold for glycan recognition (Chapter 30).
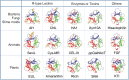
FIGURE 31.4.
Structures of β-trefoil folds of R-type lectin domains in various proteins. (Top) Structures derived from bacteria, fungi, and slime molds: AH (actinohivin in actinobacteria; PDB 4DEN), CNL (nematocidal lectin from conifer-loving mushroom Clitocybe (more...)
In view of the conserved structures consisting of variable loops and a short β-barrel, in combination with low sequence homology based on β-strands connected by loops, the ability of β-trefoil proteins to flexibly select a variety of carbohydrate partners is not surprising. β-Trefoil proteins have been divided into several groups on the basis of biological function; these include lectins, basic fibroblast growth factor (FGF) (PDB 2AXM; Chapter 38), interleukin-1 (PDB 1ILT), Kunitz-type protease inhibitors (PDB 4IHZ), and the actin cross-linking protein hisactophilin (PDB 1HCE), although they have no Q-x-W motif.
Each subdomain could conceivably have a carbohydrate-binding site; however, in most R-type lectins only one or two subdomains bind a carbohydrate ligand. Carbohydrate-binding of RCA-I and RCA-II arises from aromatic amino acids stacking against Gal/GalNAc residues and from hydrogen bonding between aa's and hydroxyl groups of carbohydrate ligands.
Diversification of R-Type Lectins in Plants
A wide variety of R-type lectins with diverse configurations, including RIP-IIs and heterotetramers such as RCA-II, have been purified from various plant tissues. Nontoxic lectins isolated from elderberry (genus Sambucus) bark include SSA (Sambucus sieboldiana agglutinin) and SNA (Sambucus nigra agglutinin UniProt Q41358). These are useful because it is the rare R-type lectin that binds strongly to α2-6-linked sialic acid–modified glycans, but not to α2-3-linked sialylated ligands. Both are heterotetramers (∼140 kDa) composed of two heterodimers, each containing an A chain (resembling ricin A chain) disulfide-bonded to a B chain (R-type lectin).
Expression of R-type lectins in roots and shoots of rice (Oryza sativa) was shown to be up-regulated in response to changing environmental conditions, based on genome informatics of Man and Gal-binding β-trefoil fold group EUL (UniProt B3SV73) found in Euonymus europaeus. Analysis of the promoter region of rice EUL genes revealed diverse stress-responsive elements. Analysis of expression profiles revealed that the genes were regulated in response to various types of stress (drought, osmotic, hormonal). Thus, β-trefoil fold lectins are involved in plant stress signaling and defense.
Molecular structures of plant proteins having R-type lectin or β-trefoil fold domains vary extensively. Amaranth (Amaranthus caudatus) is an ancient South American crop plant with high nutritional value. Amaranthin (PDB 1JLX), purified from amaranth seeds, is a homodimeric lectin (Mr 66,000) consisting of two identical tandem-repeat β-trefoil fold domains. The primary structure of the lectin is ∼20% similar to that of ricin B-chain. Amaranthin specifically recognizes Thomsen–Fredenreich (TF or T antigen) (Galβ1-3GalNAcα1-) and sialyl-T (Siaα2-3Galβ1-3GalNAcα1-) antigens. A GalNAc residue of the T-antigen disaccharide is captured by β- and γ-subdomains; thus, the mechanism of oligosaccharide binding by amaranthin differs from that of ricin B-chain and FGF (PDB 2AXM), in which a disaccharide is captured by a single subdomain.
Phylogenetic survey of plant genomes revealed that many families have amaranthin-like R-type lectin domain sequences in their polypeptides. The sequences consist either of solely lectin domains (prototype R-type lectin), and include tandem repeats, or of a combination of lectin with various functional domains (chimera-type R-type lectin). Plants use R-type lectin domain by combining it with functional domains such as “aerolysin,” a pore-forming bacterial toxin. Tandem-repeat and chimera-type genes containing an amaranthin-like R-type lectin domain are expressed in many tissues and at different developmental stages, suggesting that R-type lectins respond to various external environmental stimuli.
R-TYPE LECTINS IN FUNGI, PROTISTA, AND ANIMALS
General Properties
R-type lectins are present in fungi, protists, invertebrates, and vertebrates. Almost all of them have the same Q-x-W sequence and bind to Gal, GalNAc, and Man. Some are dimeric or oligomeric forms of simple β-trefoil subunits (prototype R-type lectin); others are tandem-repeat R-type lectins having two CRDs in the polypeptide chain. Chimeric forms have functional domains (enzymes, toxins) associated with R-type lectin, similar to those in plants and bacteria.
Fungal R-Type Lectins
Diverse forms of R-type lectins have been found in fungi (Chapter 23). Some consist solely of β-trefoil subunits. A dimeric prototype R-type lectin CNL (PDB 3NBE), from the conifer-loving mushroom C. nebularis, exerts nematocidal activity by binding to GalNAcβ1-4GlcNAc glycan expressed in nematodes. CNL and another prototype R-type lectin, BEL (PDB 4I4X), from Boletus edulis, which binds to T-antigen, inhibit growth of cultured mammalian tumor cells.
Chimeric forms in fungi include R-type lectin domains connected to tyrosinase-domain (PPO3: PDB 2Y9W), peptide N-glycanase-like-domain (MOA: PDB 2IHO), and aerolysin-like domain (LSL: PDB 1W3A). LSL from Laetiporus sulphureus (a bracket fungus) has a β-trefoil fold but not a Q-x-W motif.
Protist R-Type Lectins
Studies of R-type lectins in protists are at an early stage. One was recently found in the anaerobic parasite Entamoeba histolytica (Chapter 43), a Gal/GalNAc-binding prototype R-type lectin (PDB 6IFB) consisting of two β-trefoil subunits. In each subunit, the α- and β-subdomain functioned, respectively, to capture carbohydrates and subunits connected by a disulfide bond.
Invertebrate R-Type Lectins
Prototype and tandem-repeat R-type lectins consisting solely of β-trefoil domains have been identified in various invertebrate groups, including sponges (phylum Porifera), earthworms (Annelida) (EW29: PDB 2ZQN), bivalves (Mollusca) (UniProt A0A646QVV9), and brachiopods (Brachiopoda) (UniProt A0A646QV64). SeviL (PDB 6LF2), in mussels, binds to the trisaccharide Galβ1-3GalNAcβ1-4Gal, a component of gangliosides GM1b (Siaα2-3 Galβ1-3GalNAcβ1-4Galβ1-4Glcβ1-1Cer) and GA1 (Galβ1-3GalNAcβ1-4Galβ1-4Glcβ1-1Cer). GM1b is a target antigen of Guillain-Barré syndrome (Chapter 11), which arises from Campylobacter infection because structures of some bacterial lipopolysaccharides mimic those of the ganglioside (Chapter 20). Interaction between prototype R-type lectin and ganglioside-induced cytotoxicity against mammalian neoplastic cells through activation of MAP kinases. Another family of mussel lectins (MytiLec: PDB 3WMV; CGL: PDB 5F90) has a β-trefoil fold but not Q-x-W motif. Similar to Shiga toxins (Chapter 37), these lectins captured three Gb3 (Galα1-4Galβ1-4Glc) glycans in three carbohydrate-binding pockets within the β-trefoil fold, and thereby induced signal transduction.
Chimera-type R-type lectins connect with various functional domains in invertebrates. Pierisin in cabbage butterfly (Arthropoda, order Lepidoptera) larvae expresses R-type lectin domain at the carboxyl terminus of an ADP-ribosyltransferase domain (Uniprot Q9U8Q4) and induces apoptosis of cancer cells. This lectin domain was found to bind to glycan ligands of Gb3 and Gb4 (GalNAcβ1-3Galα1-4Galβ1-4Glc) glycosphingolipids on cancer cells termed, respectively, Pk and P antigens (Chapter 14). Clotting factor G α-subunit (Uniprot Q27082) in horseshoe crab (Arthropoda, order Crustacea) blood contained two glycoside-hydrolase domains on either side of a central R-type lectin domain. Another R-type lectin, CEL-III (PDB 2Z48), in sea cucumber (Echinodermata, Deuterostomia; Chapter 27) has both a pore-forming domain and a tandem-repeat-type R-type lectin domain and displays hemolytic effect on mammalian erythrocytes.
Almost all the configurations seen in invertebrate R-type lectins are also found in bacterial R-type lectins. β-trefoil fold is a very effective protein scaffold for biological functions. Living systems have evidently improved their survival strategies by evolutionary tinkering of the R-type lectin domain per se, or by linking it with other functional domains.
UDP-GalNAc:Polypeptide α-N-Acetyl-Galactosaminyltransferases
UDP-GalNAc: polypeptide α-N-acetylgalactosaminyltransferases (ppGalNAcTs: EC 2.4.1.41) have chimeric-form R-type lectin structures consisting of a catalytic subdomain (amino-terminal) and an R-type lectin domain (carboxy-terminal) (PDB 1HXB). Mucin-type O-glycans have a common core structure GalNAcα1-Ser/Thr (Figure 31.5), which can be modified by addition of Gal or GalNAc residues (Chapter 10). Most proteins (∼80%) that pass through the secretory apparatus have at least one Ser or Thr residue modified with α-linked GalNAc. A family of ppGalNAcTs functioning in the Golgi are responsible for modification of Ser/Thr residues.

FIGURE 31.5.
Structure and function of UDP-GalNAc:polypeptide α-N-acetylgalactosaminyltransferases (ppGalNAcTs). (A) ppGalNAcT activity with Ser/Thr-containing acceptor peptide and UDP-GalNAc as donor. Some ppGalNAcTs act preferentially on the product of this (more...)
These enzymes are widely distributed in vertebrates, invertebrates, and protists, but so far have not been found in bacteria, plants, or fungi (Chapter 26). In humans, 20 ppGalNAcT-encoding isoform genes have been studied with regard to structure, substrate specificity, and function (Chapter 6). A ppGalNAcT (Uniprot S5S833) was found in a snail that serves as an intermediate host for trematodes, the primary group of schistosomes that infect humans. The snail ppGalNAcT, in contrast to those in vertebrates, has affinity for a wide range of substrates and only one isoform.
Enzymes in this family of ppGalNAcTs have multidomain structure and are type II transmembrane proteins having the amino terminus in cytosol and carboxyl terminus on the other side of the membrane. They interact dynamically with their polypeptide substrates. In ppGalNAcT2, the R-type lectin domain interacts primarily with GalNAc on modified residues, whereas other regions of the polypeptide substrate lie in a groove next to the active site of the catalytic domain. The proximity of these two interactions positions the enzyme to bind special acceptor peptides. The parasitic protist Toxoplasma gondii (SAR supergroup, phylum Alveolata; Chapter 20) uses this enzyme to glycosylate cyst walls in the central nervous system to confer structural rigidity, thereby enabling the parasite to survive for the host's lifetime.
Vertebrate Mannose Receptors
The diversity of R-type lectin domains in vertebrates is not well-understood, relative to those in plants or invertebrates. Mannose receptors (MRs) are type I transmembrane glycoproteins and unique multifunctional chimera-form lectins consisting of an amino-terminal cysteine-rich R-type lectin domain (PDB 1DQO), a single fibronectin type II domain, and multiple C-type lectin domains (CTLDs) (Chapter 34). MR CD206 (Uniprot Q9UBG0) is expressed on hepatic endothelial cells, Kupffer cells, other epithelial cells, macrophages, and dendritic cells and functions in innate and adaptive immune systems. The domain configurations of phospholipase A2 receptor, DEC-205, and Endo180 are the same as that of CD206 (Figure 31.1), although their ligands are not glycans.
When CD206 dimerizes on cell membrane, the R-type lectin domain binds to sulfated glycans. Pituitary hormones are captured by binding of this domain to 4-SO4-GalNAcβ1-R residues, 3-O-sulfated Gal, 3-O-sulfated Lex, or 3-O-sulfated Lea for clearance from the bloodstream. CTLDs recognize surface mannose-rich glycoconjugates on Candida albicans, Pneumocystis carinii, Mycobacterium tuberculosis, Klebsiella pneumoniae, and Leishmania donovani (Chapter 43). CD206 is considered a pathogen-associated molecular pattern (PAMP) that functions in clathrin-dependent endocytosis and in phagocytosis of nonopsonized microbes. MRs also play roles in adaptive immunity through their ability to deliver antigens to major histocompatibility class II compartments. Their R-type lectin domains facilitate binding to dendritic cells that express sulfated glycan-containing ligands such as sialoadhesin and CD45.
MICROBIAL R-TYPE LECTINS
General Properties
Most of the known R-type lectin domains are found in bacterial lectins, enzymes, and toxins. Genes encoding R-type lectins have been observed in Archaea and viruses, evidently reflecting evolution from bacteria to eukaryotes. Microbial genes of the original R-type lectins may have been transmitted to eukaryotes; alternatively, lectins may have arisen through convergent evolution in the two groups.
Bacterial R-Type Lectins
Actinohivin (AH; PDB 4DEN), a prototype R-type lectin in bacteria, consists of 114-aa polypeptides isolated from actinobacteria (genus Actinomyces). It binds to high mannose-type oligosaccharides, commonly found on the viral envelope GP120 of human immunodeficiency virus 1 (HIV-1). AH displays strong anti-HIV activity against clinically isolated viral strains (Chapter 42).
R-type lectin domains are found in many bacterial enzymes and toxins. An example in Streptomyces is the enzyme endo-β1-4-xylanase (Xyn10A: EC 3.2.1.8; PDB 1ISX). Xyn10A acts as a glycoside hydrolase to catalyze cleavage of β1-4 xylose and can bind to xylan and a variety of soluble sugars, including Gal, lactose, and xylo- and arabino-oligosaccharides.
Pore-forming domains are found in exotoxins produced by pathogenic bacteria that hemolyze erythrocytes. A monomeric lysin domain comprised of β-sheets oligomerizes like a piece of fabric tube to create pores on the cell wall. Aerolysin (Chapter 12) and hemolysin are bacterial toxins having R-type lectin domains. Many eukaryotes (plants, fungi, invertebrates) have aerolysin-like domains. Hemolysin (UniProt P09545) of Vibrio cholerae has a β-prism domain with jacalin (jackfruit lectin) structure, as well as R-type lectin domain.
Botulinum neurotoxin (BoNT) from Clostridium botulinum has extremely high toxicity (LD50: 0.001 µg/kg) (Chapter 37). A component of BoNT termed hemagglutinin HA1 (PDB 3AH2) is a tandem-repeat-type dimeric R-type lectin subunit that binds to Gal at the carboxy-terminal subdomain. HA2, a subunit with β-trefoil fold, connects to the amino-terminal subdomains of two HA1s and to the jelly-roll-like β-sandwich fold subunit HA3 (PDB 2ZOE) (Chapter 32). HA1 helps orient BoNT to target intestinal cells by binding to Gal or Sia in gangliosides (e.g., GT1b) (Chapter 11) or cell-surface glycans.
MTX (UniProt Q03988), a mosquitocidal toxin found in Bacillus sphaericus, contains an ADP-ribosyltransferase domain and four R-type lectin domains, arranged in a structural configuration similar to that of pierisin in cabbage butterfly larvae. Subunit forms of MTX are the same as those of cholera toxin and ricin (i.e., they consist of A-subunit [toxic enzyme] and B-subunit [lectin]).
Archaeal and Viral R-Type Lectins
R-type β-trefoil lectins have been identified in numerous species in the families Halobacteriaceae and Natrialbaceae (class Haloarchaea), whose cell walls have mucin-containing glycoproteins (Chapter 22). Based on successful experimental culture of an archaeal strain, a model was proposed in 2020 for eukaryogenesis from Archaea. An intriguing possibility is that domain Archaea is the link for R-type lectins between domains Bacteria and Eukarya.
Type-C and type-D BoNTs have been produced from the genome of a Clostridium bacteriophage. Were R-type lectin genes somehow produced by viruses? Metagenomic analyses indicate the presence of R-type lectin genes (Uniprot Q5UPX2) in the viral family Mimiviridae (Chapters 8 and 20), and phylum Nucleocytoviricota. Recent surveys reveal that the genus Mimivirus is distributed worldwide in oceans as well as on land. Extensive future studies of comparative expression levels of viral R-type lectin genes will help clarify relationships between glycan-codes and specific environments in the world's ecosystems.
PERSPECTIVES
The term “bricolage” refers to creation of novel tools in primitive societies in which availability of materials was limited. Creation of such tools may be based on tinkering with frameworks comprising limited sets of products and techniques used previously for other purposes. Analogously, modification and/or combination of genes in different groups of organisms may generate molecules useful for novel environmental responses. The β-trefoil fold structure, characteristic of the R-type lectin superfamily, is evidently a core framework in living systems that arose via bricolage and has evolved to play key roles in a variety of biochemical processes, including carbohydrate binding.
Discovery of new R-type lectins is ongoing. The ancestor of this superfamily is not yet clear, but it is found in members of all biological domains. The origins and evolution of these lectins are therefore of interest to scientists in many fields. The presence of β-trefoil folding in proteins may reflect either convergent or divergent evolution. Elucidating the physiological functions of various R-type lectins will require identification of their endogenous ligands and the specific environmental stimuli that trigger their expression. Increasing knowledge of R-type lectin structure and function will enhance our understanding of lectin-glycan interactions in living systems and facilitate biotechnological applications of these lectins.
ACKNOWLEDGMENTS
The authors acknowledge contributions to previous versions of this chapter by Marilynn E. Etzler, helpful comments and suggestions by Sneha S. Komath, support in preparation of Figure 31.4 by Kenichi Kamata, and English editing of the manuscript by Stephen Anderson.
FURTHER READING
- Murzin AG, Lesk AM, Chothia C. 1992. β-Trefoil fold: patterns of structure and sequence in the Kunitz inhibitors interleukins-1β and -1α and fibroblast growth factors. J Mol Biol 223: 531–543. doi:10.1016/0022-2836(92)90668-a [PubMed: 1738162] [CrossRef]
- Stirpe F, Barbieri L, Battelli MG, Soria M, Lappi DA. 1992. Ribosome-inactivating proteins from plants: present status and future prospects. Biotechnology 10: 405–412. doi:10.1038/nbt0492-405 [PubMed: 1368484] [CrossRef]
- Lord JM, Roberts LM, Robertus JD. 1994. Ricin: structure, mode of action, and some current applications. FASEB J 8: 201–208. doi:10.1096/fasebj.8.2.8119491 [PubMed: 8119491] [CrossRef]
- Hazes B. 1996. The (QxW)3 domain: a flexible lectin scaffold. Protein Sci 5: 1490–1501. doi:10.1002/pro.5560050805 [PMC free article: PMC2143495] [PubMed: 8844840] [CrossRef]
- Hirabayashi J, Dutta SK, Kasai K. 1998. Novel galactose-binding proteins in Annelida. Characterization of 29-kDa tandem repeat-type lectins from the earthworm Lumbricus terrestris. J Biol Chem 273: 14450–14460. doi:10.1074/jbc.273.23.14450 [PubMed: 9603958] [CrossRef]
- Leteux C, Chai W, Loveless RW, Yuen CT, Uhlin-Hansen L, Combarnous Y, Jankovic M, Maric SC, Misulovin Z, Nussenzweig MC, Feizi T. 2000. The cysteine-rich domain of the macrophage mannose receptor is a multispecific lectin that recognizes chondroitin sulfates A and B and sulfated oligosaccharides of blood group Lewis(a) and Lewis(x) types in addition to the sulfated N-glycans of lutropin. J Exp Med 191: 1117–1126. doi:10.1084/jem.191.7.1117 [PMC free article: PMC2193175] [PubMed: 10748230] [CrossRef]
- Matsushima-Hibiya Y, Watanabe M, Hidari KI, Miyamoto D, Suzuki Y, Kasama T, Kasama T, Koyama K, Sugimura T, Wakabayashi K. 2003. Identification of glycosphingolipid receptors for pierisin-1, a guanine-specific ADP-ribosylating toxin from the cabbage butterfly. J Biol Chem 278: 9972–9978. doi:10.1074/jbc.m212114200 [PubMed: 12645583] [CrossRef]
- Uchida T, Yamasaki T, Eto S, Sugawara H, Kurisu G, Nakagawa A, Kusunoki M, Hatakeyama T. 2004. Crystal structure of the hemolytic lectin CEL-III isolated from the marine invertebrate Cucumaria echinata: implications of domain structure for its membrane pore-formation mechanism. J Biol Chem 279: 37133–37141. doi:10.1074/jbc.m404065200 [PubMed: 15194688] [CrossRef]
- Nakamura T, Tonozuka T, Ide A, Yuzawa T, Oguma K, Nishikawa A. 2008. Sugar-binding sites of the HA1 subcomponent of Clostridium botulinum type C progenitor toxin. J Mol Biol 376: 854–867. doi:10.2210/pdb2ehn/pdb [PubMed: 18178224] [CrossRef]
- Broom A, Doxey AC, Lobsanov YD, Berthin LG, Rose DR, Howell PL, McConkey BJ, Meiering EM. 2012. Modular evolution and the origins of symmetry: reconstruction of a three-fold symmetric globular protein. Structure 20: 161–171. doi:10.1016/j.str.2011.10.021 [PubMed: 22178248] [CrossRef]
- Fujii Y, Dohmae N, Takio K, Kawsar SMA, Matsumoto R, Hasan I, Koide Y, Kanaly RA, Yasumitsu H, Ogawa Y, et al. 2012. A lectin from the mussel Mytilus galloprovincialis has a highly novel primary structure and induces glycan-mediated cytotoxicity of globotriaosylceramide-expressing lymphoma cells. J Biol Chem 287: 44772–44783. doi:10.1074/jbc.m112.418012 [PMC free article: PMC3531790] [PubMed: 23093409] [CrossRef]
- Abhinav KV, Samuel E, Vijayan M. 2016. Archeal lectins: an identification through a genomic search. Proteins 84: 21–30. doi:10.1002/prot.24949 [PubMed: 26492087] [CrossRef]
- Hasan I, Gerdol M, Fujii Y, Rajia S, Koide Y, Yamamoto D, Kawsar SMA, Ozeki Y. 2016. cDNA and gene structure of MytiLec-1, a bacteriostatic R-type lectin from the Mediterranean mussel (Mytilus galloprovincialis). Mar Drugs 14: 92. doi:10.3390/md14050092 [PMC free article: PMC4882566] [PubMed: 27187419] [CrossRef]
- Dang L, Rougé P, Van Damme EJM. 2017. Amaranthin-like proteins with aerolysin domains in plants. Front Plant Sci 8: 1368. doi:10.3389/fpls.2017.01368 [PMC free article: PMC5554364] [PubMed: 28848572] [CrossRef]
- De Schutter K, Tsaneva M, Kulkarni SR, Rougé P, Vandepoele K, Van Damme EJM. 2017. Evolutionary relationships and expression analysis of EUL domain proteins in rice (Oryza sativa). Rice (NY) 10: 26. doi:10.1186/s12284-017-0164-3 [PMC free article: PMC5449364] [PubMed: 28560587] [CrossRef]
- Liao J, Chien CH, Wu H, Huang K, Wang I, Ho M, Tu I, Lee I, Li W, Shih Y, et al. 2017. A multivalent marine lectin from Crenomytilus grayanus possesses anti-cancer activity through recognizing globotriose Gb3. J Am Chem Soc 138: 4787–4795. doi:10.1021/jacs.6b00111.s001 [PubMed: 27010847] [CrossRef]
- Terada D, Voet ARD, Noguchi H, Kamata K, Ohki M, Addy C, Fujii Y, Yamamoto D, Ozeki Y, Tame JRH, Zhang KYJ. 2017. Computational design of a symmetrical β-trefoil lectin with cancer cell binding activity. Sci Rep 7: 5943. doi:10.1038/s41598-017-06332-7 [PMC free article: PMC5517649] [PubMed: 28724971] [CrossRef]
- Franke H, Scholl R, Aigner A. 2019. Ricin and Ricinus communis in pharmacology and toxicology-from ancient use and “Papyrus Ebers” to modern perspectives and “poisonous plant of the year 2018”. Naunyn Schmiedebergs Arch Pharmacol 392: 1181–1208. doi:10.1007/s00210-019-01691-6 [PubMed: 31359089] [CrossRef]
- Hu Z, Wang Y, Cheng C, He Y. 2019. Structural basis of the pH-dependent conformational change of the N-terminal region of human mannose receptor/CD206. J Struct Biol 208: 107384–107391. doi:10.1016/j.jsb.2019.09.001 [PubMed: 31491467] [CrossRef]
- Fujii Y, Gerdol M, Kawsar SMA, Hasan I, Spazzali F, Yoshida T, Ogawa Y, Rajia S, Kamata K, Koide Y, et al. 2020. A GM1b/asialo-GM1 oligosaccharide-binding R-type lectin from purplish bifurcate mussels Mytilisepta virgata and its effect on MAP kinases. FEBS J 287: 2612–2630. doi:10.1111/febs.15154 [PMC free article: PMC7317968] [PubMed: 31769916] [CrossRef]
- Ismaya WT, Tjandrawinata RR, Dijkstra BW, Beintema JJ, Nabila N, Rachmawati H. 2020. Relationship of Agaricus bisporus mannose-binding protein to lectins with β-trefoil fold. Biochem Biophys Res Commun 527: 1027–1032. doi:10.1016/j.bbrc.2020.05.030 [PubMed: 32439171] [CrossRef]
- Kamata K, Mizutani K, Takahashi K, Marchetti R, Silipo A, Addy C, Park S-Y, Fujii Y, Fujita H, Konuma T, et al. 2020. The structure of SeviL, a GM1b/asialo-GM1 binding R-type lectin from the mussel Mytilisepta virgata. Sci Rep 10: 22102. doi:10.1038/s41598-020-78926-7 [PMC free article: PMC7744527] [PubMed: 33328520] [CrossRef]
- Khan F, Kurre D, Suguna K. 2020. Crystal structures of a β-trefoil lectin from Entamoeba histolytica in monomeric and a novel disulfide-bond-mediated dimeric forms. Glycobiology 30: 474–488. doi:10.1093/glycob/cwaa001 [PubMed: 31967310] [CrossRef]
- Review R-type Lectins.[Essentials of Glycobiology. 2009]Review R-type Lectins.Cummings RD, Etzler ME. Essentials of Glycobiology. 2009
- Review R-Type Lectins.[Essentials of Glycobiology. 2015]Review R-Type Lectins.Cummings RD, L. Schnaar R. Essentials of Glycobiology. 2015
- Carbohydrate recognition factors of the lectin domains present in the Ricinus communis toxic protein (ricin).[Biochimie. 2006]Carbohydrate recognition factors of the lectin domains present in the Ricinus communis toxic protein (ricin).Wu JH, Singh T, Herp A, Wu AM. Biochimie. 2006 Feb; 88(2):201-17. Epub 2005 Aug 18.
- C-type lectin-like carbohydrate recognition of the hemolytic lectin CEL-III containing ricin-type -trefoil folds.[J Biol Chem. 2007]C-type lectin-like carbohydrate recognition of the hemolytic lectin CEL-III containing ricin-type -trefoil folds.Hatakeyama T, Unno H, Kouzuma Y, Uchida T, Eto S, Hidemura H, Kato N, Yonekura M, Kusunoki M. J Biol Chem. 2007 Dec 28; 282(52):37826-35. Epub 2007 Oct 31.
- Evolution of tetrameric lectin Ricinus communis agglutinin from two variant groups of ricin toxin dimers.[Eur J Biochem. 1998]Evolution of tetrameric lectin Ricinus communis agglutinin from two variant groups of ricin toxin dimers.Hegde R, Podder SK. Eur J Biochem. 1998 Jun 15; 254(3):596-601.
- R-Type Lectins - Essentials of GlycobiologyR-Type Lectins - Essentials of Glycobiology
Your browsing activity is empty.
Activity recording is turned off.
See more...