NCBI Bookshelf. A service of the National Library of Medicine, National Institutes of Health.
Probe Reports from the NIH Molecular Libraries Program [Internet]. Bethesda (MD): National Center for Biotechnology Information (US); 2010-.
Cancer stem cells (CSCs), which drive tumor growth, are known to be resistant to standard chemotherapy and radiation treatment. This raises a significant unmet need to find therapies that can target CSCs within tumors because these cells are responsible for recurrence, the primary cause of patient mortality. However, one of the technical challenges of working with CSCs is that they are not stable outside the tumor environment and are not easy to grow in culture media. Hence, stable sibling cell lines that were induced into epithelial-to-mesenchymal transdifferentiation (EMT) to stably propagate CSC-enriched populations were used to screen a library of 300,718 compounds from the Molecular Libraries Small Molecule Repository (MLSMR). Several classes of selective inhibitors of CSCs were identified. The use of isogenic control cell lines for the secondary validation assays minimized the probability of false hits advancing along the critical path to probe development. Of these, 19 compounds were chosen based on their selectivity, potency, and chemical tractability and were retested in the primary screen and secondary assays. Three scaffolds were prioritized to develop potential probes. One of these compounds (ML243) displayed greater than 32-fold selective inhibition of the breast CSC-like cell line (HMLE_shECad) over the control cell line (HMLE_shGFP). The probe (ML243) was screened against a panel of 68 targets that are commonly used in drug discovery for lead profiling and found active in only one assay.
Assigned Assay Grant No.: 1 R03 MH089663-01
Screening Center Name and PI: Broad Institute Probe Development Center, Stuart Schreiber
Chemistry Center Name and PI: Broad Institute Probe Development Center, Stuart Schreiber
Assay Submitter and Institution: Eric Lander, Broad Institute and Piyush Gupta, Whitehead Institute
PubChem Summary Bioassay Identifier: AID 2721
Probe Structure & Characteristics
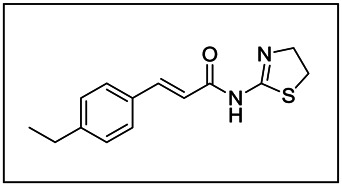
ML243
CID/ML# | Target Name | EC50 (μM) [SID, AID] | Anti-target Name | EC50 (μM) [SID, AID] | Fold Selective | Secondary Assay: EC50 (nM) [SID, AID] |
---|---|---|---|---|---|---|
CID 50910523/ML243 | Breast Cancer Stem Cell-like cell | 2.0 μM [SID 112208912, AID 504535] | Mammary Epithelial Cell | 63.98 μM [SID 112208912, AID 504533] | 32 | Tumorsphere, single point, Inactive [SID 112208912, AID 504859] |
1. Recommendations for Scientific Use of the Probe
There is an obvious unmet need for developing compounds that are selectively lethal to breast cancer stem cell (CSCs). Breast CSCs are thought to be responsible for cancer recurrence and resistance to chemotherapeutic treatments. Developing probes against breast CSCs enables researchers to gain a better perspective and a pathway to targeting these cells in the clinic.
Nine small molecules have previously been identified to selectively inhibit breast CSCs. Only one molecule, salinomycin, has been identified to selectively inhibit this particular breast CSC-like cell line (HMLE_shECad). Although it has a potency of approximately 1 μM against this cell line, it should be noted that salinomycin also shows toxicity against the control cell line (HMLE_shGFP) at 10 μM. Therefore, the goal of this project is to find a potent probe with less toxicity liability to control cell lines. In addition to the clinical value, these probes would have the potential to be used for novel target identification.
The current body of work identifies numerous genes whose expression is modified by treatment with the probe (CID 50910523/ML243 — referred to as ML243 throughout this document). Treatment of the HMLE-shECad cell line identified gene expression changes in several membrane associated proteins (see Figure 4) and endosomal cycling proteins, suggesting a role in regulating cycling proteins to the membrane. Furthermore, this probe (ML243) is currently being optimized for use in binding studies with the intent of identifying direct molecular targets within the cell. Furthermore, the chemical probe may also be used to investigate pathways developing CSCs, lineage, self-renewal, and other basic research to develop a better understanding of Breast CSCs.
2. Materials and Methods
See subsections for a detailed description of the materials and methods used for each assay.
2.1. Assays
Primary HTS Using CellTiter-Glo (AID 2717)
HMLE_shECad cells were prepared as previously described [7].
Procedures: Briefly, HMLE cells expressing either shRNA targeting E-cadherin (shECad) were propagated in 1:1 mixture of 10% fetal bovine serum (FBS; HyClone), 1% Penicillin/Streptomycin (Pen/Strep; Cellgro), 1% Glutamax-1 (Invitrogen), 70 nM Hydrocortisone (Sigma), 12 μg/mL Insulin (Sigma), 50 μg/ml Gentamicin (Sigma), 12.5 μg/mL Plasmocin (InVivogen), 10 ng/mL EGF in DMEM (Cellgro), and Mammary Epithelial Cell Growth Medium (MEGM complete medium; Lonza, Basel, Switzerland) at 37ºC, 5% CO2. For screening, the cells were counted and resuspended in complete media without serum. Next, 2,000 cells in 50 νL were plated per well in white, tissue culture-treated, 384-well plates (Corning). The cells were incubated at 37ºC, 5% CO2 for at least 4 hours and pinned with 100 nl of compounds. The cells were incubated approximately 72 hours, then 30 μL of CellTiter-Glo (Promega) diluted 1:3 with PBS was added to the well. The plates were read using the EnVision (PerkinElmer; Luminescence 0.1 sec/well) after 12 minutes.
Primary Retest (AID 449748)
Repeat of the primary screen at dose using CellTiter-Glo.
Primary Cell Viability Protocol with HMLE_shECad Cells Using CellTiter-Glo (AID 493226, AID 493176, AID 504449, AID 504535, AID 504667, AID 504788)
HMLE_shECad cells were prepared as previously described [7].
Procedures: Briefly, HMLE cells expressing either shRNA targeting E-cadherin (shECad) were propagated in 1:1 mixture of 10% fetal bovine serum (FBS; HyClone), 1% Penicillin/Streptomycin (Pen/Strep; Cellgro), 1% Glutamax-1 (Invitrogen), 70 nM Hydrocortisone (Sigma), 12 μg/mL Insulin (Sigma), 50 μg/mL Gentamicin (Sigma), 12.5 μg/mL Plasmocin (InVivogen), 10 ng/mL EGF in DMEM (Cellgro) and Mammary Epithelial Cell Growth Medium (MEGM complete medium; Lonza, Basel, Switzerland) at 37ºC, 5% CO2. For screening, the cells were counted and resuspended in complete media without serum. Next, 2,000 cells in 50 μL were plated per well in white, tissue culture-treated, 384-well plates (Corning). The cells were incubated at 37ºC, 5% CO2 for at least 4 hours and pinned with 100 nl of compounds. The cells were incubated approximately 72 hours, then 30 μL of CellTiter-Glo (Promega) diluted 1:3 with PBS was added to the well. The plates were read using the EnVision (PerkinElmer; Luminescence 0.1 sec/well) after 12 minutes.
Secondary Cell Viability Protocol with HMLE_shGFP Cells Using CellTiter-Glo (AID 504325, AID 493196, AID 504450, AID 504533, AID 504666, AID 504789)
HMLE_shGFP cells were prepared as previously described [7].
Assay Provider Cell Viability Protocol with CellTiter-Glo
HMLE_shTWIST cells were prepared as previously described [7].
Secondary Orthogonal Assay for In Vitro Inhibition of Tumorspheres with Sum159 Cells (AID 504623, AID 504623)
Sum159 cells were provided by the Assay Provider as previously described [7].
Procedures: Tumorsphere assays were performed as previously described [8] with minor changes. Sum159 cells were propagated in 5% FBS (HyClone), 1% Pen/Strep, 1% Glutamax-1, 12 μg/mL Insulin, 50 μg/mL Gentamicin in F12/DMEM (Cellgro). Next, 96-well, ultra-low adhesion plates (Costar) were plated with 100 μL media respective to cell type. Then, 400 nL of compounds were pinned into the media. The cells were harvested, counted, and resuspended in their propagation media with 1% methylcellulose (ES-CultM3120, Stem Cell Technologies). Then, 100 μL of resuspended cells were added to the plates containing media with compound for a final count of 2000 cells/well in 200 μL with 0.5% methylcellulose. Tumorspheres were allowed to form for 9 days incubated at 37°C, 5% CO2. Tumorspheres were imaged using a 2X objective on the ImageXpress Micro (Molecular Devices, Sunnyvale, CA). Cell clusters greater than 100 μM in diameter were identified using MetaXpress software (version 3.1; Molecular Devices).
Gene Expression Assays with HMLE_shECad and HMLE_shGFP Cells
Triplicate samples of HMLE_shECad and HMLE_shGFP cells were treated with vehicle (DMSO) or compound at an IC50 dose for 24 hours prior to isolation of RNA. Total RNA was isolated using the RNeasy Protect Mini Kit (Qiagen). Quality control (QC) processing of the RNA samples and the gene expression analysis were performed by the Genome Analysis Platform (GAP) at the Broad Institute.
Procedures: Briefly, RNA samples were analyzed for quality using Aglient Bioanalyzer Chips. cRNA synthesis from the total RNA samples passing QC was prepared for analysis on a HumanHT-12 Expression BeadChip (Illumina, San Diego, CA) according to the manufacturer’s instructions. Normalization of the raw gene expression data, QC checks, and analyses were done with GenomeStudio (version 2010.3; Illumina, San Diego, CA). All of the samples were run in triplicate; the data set was normalized using the quantile module. After the samples were normalized, gene expression was compared and those with a significant difference (p>0.005) and 1.25-fold increase in expression were selected.
2.2. Probe Chemical Characterization
After preparation as described in Section 2.3, the probe (ML243) was analyzed by UPLC, 1H and 13C NMR spectroscopy, and high-resolution mass spectrometry. The data obtained from NMR and mass spectroscopy were consistent with the structure of the probe, and UPLC indicated an isolated purity of >95%.
The solubility of the probe (ML243) was experimentally determined to be 11.5 μM in Phosphate buffered saline (PBS, pH 7.4, 23°C) solution. Plasma protein binding (PPB) was determined to be 96.6% bound in human plasma. The probe is stable in human plasma, with approximately 97.1% remaining after a 5-hour incubation period. The compound was found to be stable in glutathione (GSH) with 82% remaining after 48 hours.
The stability of the probe (ML243) in PBS (0.1% DMSO) was measured over 48 hours. We noticed that the concentration of the probe steadily increased over 48 hours to about 900% (data not shown). Since the solubility of the probe in PBS is low, we believe that the gradual increase in concentration is the direct result of more compound dissolving in PBS over time. In other words, when we performed the PBS stability assay under the recommended conditions; we measured the kinetic solubility of the probe in PBS and not its stability. Thus, we decided to determine the total amount of the probe present in the well after the probe was treated with PBS alone for a given length of time. We added acetonitrile at various time points to wells containing the probe in PBS and measured the total amount of the probe. This result is shown in Figure 1. From these results, the probe seems to be stable in PBS since more than 75% is still present after 48 hours of incubation.

Figure 1
Stability Data for the Probe (ML243) in PBS Buffer (pH 7.4, 23°C) Plus Acetonitrile over 48 Hours.
Table 1Summary of Known Probe Properties in PubChem
IUPAC Chemical Name | (E)-N-(4,5-dihydro-1,3-thiazol-2-yl)-3-(4-ethylphenyl)prop-2-enamide |
---|---|
PubChem CID | CID 50910523 |
Molecular Weight | 260.35464 [g/mol] |
Molecular Formula | C14H16N2OS |
H-Bond Donor | 1 |
H-Bond Acceptor | 2 |
Rotatable Bond Count | 4 |
Exact Mass | 260.098334 |
Topological Polar Surface Area | 66.8 |
2.3. Probe Preparation
The probe (ML243) was synthesized from (E)-3-(4-ethylphenyl)acrylic acid and 4,5-dihydrothiazol-2-amine in one step (see Scheme 1). Coupling of (E)-3-(4-ethylphenyl)acrylic acid and 4,5-dihydrothiazol-2-amine in the presence of EDCI and DMAP provided the probe (E)-N-(4,5-dihydrothiazol-2-yl)-3-(4-ethylphenyl)acrylamide in 40% yield.

Scheme 1
Synthesis of the Probe (ML243).
3. Results
3.1. Dose Response Curves for Probe
Figure 2Dose-dependent Activity of the Probe (ML243) in Target and Counterscreen Assays
Primary screen from dry powders on HMLE_shECad (IC50 = 2.0 μM) (AID 504535, 504667) (A); control cell line HMLE_shGFP (63.98 μM) Toxicity (AID 504533, 504666) (B).
3.2. Cellular Activity
In order to confirm results obtained by the probe Screening Center, several additional experiments were conducted by the Assay Provider. The probe (ML243) and five additional compounds (CID 49843106, CID 5416418, CID 897475, CID 47099517, CID 896331) were investigated for their effects on viability in HMLE_shECad and HMLE_shGFP cell lines. Although different absolute IC50 values were obtained, ML243 had the best potency and greatest selectivity of all the compounds tested (Figure 3, Table 2).
Table 2
Data from Additional Assays.

Figure 3Dose Inhibition Assay of HMLE_shGFP, HMLE_shECad, and HMLE_shTwist by the Probe (ML243)
Two additional transformed cell lines, HMLE_shTWIST and MDA231, were used to corroborate the findings. HMLE_shTWIST, a second upstream model of EMT-induced breast CSC-like cells, was also inhibited by the probe (ML243) (Figure 3, Table 2). Although ML243 was not as potent against HMLE_shTwist, the probe was selective towards the EMT-induced cell lines over the control (Figure 3, Table 2). This corroborates that the compound is not specific to the generated cell line HMLE_shECad and can inhibit other breast cancer stem cell-like cells.
Furthermore, ML243 probe activity was also tested against a breast cancer cell line, MDA231 (Table 2). ML243 was weakly toxic to the cancer cell line. This may be due to the fact that MDA231 cell line contains a mixture of Breast CSCs, as well as more differentiated cells, which the compound does not target. It is possible that the addition of a chemotherapeutic drug, which targets the non-Breast Cancer Stem Cell population, would be able to fully eradicate the mixed population cancer line. Further study will be required to determine if this probe could work in conjunction with other chemotherapeutic drugs for a better outcome..
Although the probe (ML243) did not significantly inhibit tumorsphere formation, CID 6262187 did inhibit tumorsphere growth. As we observed in Scaffold 1, CID 6262187 is a nonselective compound and is toxic to both cell lines. Targeting both cell lines appears to be required for inhibiting SUM159 tumorsphere. Taken together, the probe (ML243) clearly meets the established probe criteria in whole cells specified for this project (Figures 2 and 3 and Table 3).
Table 3
Comparison of the Probe (ML243) to Project Criteria.
3.3. Profiling Assays
In order to gain insight into the possible mechanism or signaling pathways that the probe (ML243) could be targeting, gene expression studies were conducted. HMLE_shECad and HMLE_shGFP cells were treated with 2 μM (IC50) of the probe (ML243) for 24-hours in triplicate. In addition, control samples were included where DMSO was added for 24-hours. cRNA synthesis from the total RNA samples were analyzed on a Human HT-12 Expression BeadChip (Illumina). All samples passed QC standards for gene expression and hybridization.
Samples were normalized and the gene expression of the triplicate samples were compared using Comparitive Selection Module in GenePattern (Open source: http://genepattern.broadinstitute.org/). Gene expression that had p≤ 0.005 and a change of at least 125% (±25%) change in expression were selected. First, the control conditions between the cell lines were compared and 624 genes were differentially expressed (Figure 4A). Comparable results were observed previously [9]. Moreover, many of the genes confirmed the results observed by the Weinberg laboratory using these cell lines [10]. Specifically, of the 19 genes indicated by Onder et al. as statistically significantly different, 14 repeated in our assay (Figure 4A; [9, 10]). Moreover, Onder et al. identified several proteins with altered expression, including vimentin, beta-Catenin, gamma-Catenin, CK-8, and fibronectin [10]. We observed similar changes in our gene expression assay, except for fibronectin, where we did not observe any change in gene expression. However, gene expression does not always correlate with alterations in protein expression. Further characterization is required to determine if those results varied.
Next, we compared differences in gene expression after 24-hour treatment with the probe compound (ML243). Twenty-five genes had altered expression levels in HMLE_shECad cells (Figure 4B). Most of the genes that were altered were surface expression proteins, such as Sodium/sulfate transporter (SLC13A4) [11], alpha-1D adrenergic receptor (ADRA1D) [12], phospholipid transfer protein (PLTP) [13], orphan GPCR (GPR162) [14], Ephrin-B3 (EFNB3) [15], Interleukin 7 receptor (IL7R), and phosphoribosyl pyrophosphate amidotransferase (PPAT). While it is possible that the ML243 is altering these genes directly causing a change in their expression profiles, it is also likely that the probe is altering transport or recycling mechanisms.
ML243 also had effects in the control cell line HMLE_shGFP. When comparing DMSO treated vs. probe treated cells, twenty-seven genes had altered expression (Figure 4C). Several of these are membrane associated proteins, like transmembrane protein 59-like (TMEM59L), and TNF receptor-associated protein (TRAP1). In addition, VPS36, a vacuole protein sorting gene involved in endosomal sorting [16] was identified. Only the gene Nupr1 (P8), nuclear protein transcriptional regulator 1, was differentially expressed in both HMLE_shECad and HMLE_shGFP cell lines after treatment. This gene expression is regulated by cellular stressors and is induced by activating transcription factor4, ATF4 [17], which was found to be altered in expression with treatment with Probe2 (ML245).
A recent paper found that Salinomycin inhibited the WNT-signaling pathway [18]. Mutations in the Wnt/beta-catenin signaling cascade has been implicated in many cancers, and the authors suggest that targeting of Wnt signaling pathway causes toxicity in Breast Cancer Stem cells [18]. After 24-hour treatment with Salinomycin, there was a significant decrease in expression of Wnt5b and Dickkopf-related protein 1 (DKK1), which are known Wnt pathway members (Data not shown). However, no Wnt pathway genes were variably expressed after 24-hr treatment with ML243. The probe identified in this report may be working via a different pathway. Alternatively, ML243 may target the Wnt pathway at the protein level and not alter RNA expression levels.
Further study is required to understand the role of the probe (ML243) in the HMLE_shECad cells and breast CSCs. In parallel, we have identified two additional chemical scaffolds (Probe 1, submitted April 2011; Probe 2, submitted Sept. 2011) that will complement Probe 3 described in this report. We conducted similar gene expression studies with all of these probes, which may enable us to further identify and validate potential targets for breast CSCs.
The probe (ML243) was screened against a panel of 68 targets that are commonly used in drug discovery for lead profiling. The assays were done by Ricerca Biosciences, LLC and include targets from various areas such as, GPCRs, ion channels, and transporters. ML243 was only active in one of the 68 assays, where ML243 blocked radio labeled ligand to the Adenosine A2A Receptor by 51% at 10μM.
4. Discussion
4.1. Comparison to Existing Art and How the New Probe is an Improvement
The current state of the art for the probe molecule salinomycin exhibits a potency of about 1 μM against breast CSCs [7]. However, it should be noted that salinomycin shows toxicity against the control cell line (HMLE_shGFP) at 10 μM. Whereas the new probe (ML243) exhibits a similar potency at 2.0 μM, this probe is more selective and more attractive structurally allowing for rapid analog synthesis.
In the investigation of prior art relevant to the breast cancer stem cell project, the following databases were used: SciFinder, Entrez, PubMed, PubChem, PatentLens. Abstracts were obtained for all references returned and were analyzed for relevance to the current project. The searches were performed on, and are current as of, April 11, 2011.
Literature and patent searches revealed nine small molecules capable of selectively inhibiting breast CSCs (see Figure 5). It is important to note that salinomycin is the only small molecule shown to be effective in the cell line HMLE_shECad used in the primary assay. The effectiveness of salinomycin in HMLE_shECad cells was determined by the assay provider for this project. Salinomycin was shown to provide a reduction in the proportion of CSCs with an IC50 value of approximately 1 μM against HMLE_shECad with approximately 10-fold selectivity over the control HMLE_shGFP [7]. In addition, ex vivo and in vivo treatment of mice with intraperitoneal injection of salinomycin inhibited mammary tumor growth and increased differentiation of epithelial tumor cells. To date, this is the only report of selectively active compounds against the HMLE_shECad cell line.
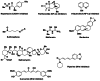
Figure 5
Known Small Molecules Inhibitors of Breast Cancer Stem Cells.
There has been a recent increase in the research of selective killing of breast CSCs. Though interesting, the cells used in this report are not susceptible to these agents. This may be because these HMLE_shECad cells were an independent, stable cell line. Other studies have used mixed cultures where Breast CSCs may be dependent on cofactors from other populations of cells. In one approach, researchers targeted the breast CSC pathways involved in self-renewal and survival. These pathways include NF-κβ, Notch, Hedgehog, and Wnt. The NF-κβ inhibitors parthenolide and 8-quinolinol (8Q) were reported by Zhou et al. to inhibit breast CSC-dependent MCF7 sphere cell growth selectively over bulk MCF7 cell growth [20]. They reported that parthenolide and pyrrolidinedithiocarbamate inhibited sphere MCF7 cell growth by 33% and 51%, respectively, and showed no obvious growth inhibition of bulk MCF7 cells. This work was followed by a 2009 publication, in which Zhou et al. reported that 8Q inhibited the sphere cell proliferation by 86% at 5 μM and inhibited the bulk cells by 30% [21]. 8Q also showed some antitumor activity in both MCF7 and MDAMB-435 (breast cancer cell line) xenograft models, but it had a much better effect when combined with paclitaxel than either agent administered alone. Kakarala et al. reported that curcumin and piperine, respectively, inhibited Wnt signaling, ALDH+ cells (breast CSC biomarker) and mammosphere formation by 50% at 5 μM and completely at 10 μM [22]. In 2010, Li et al. showed that sulforaphane down-regulated the Wnt-pathway and could inhibit ALDH+ cells in vitro by 65–80% at 1–5 μM, but had minimal effect on the population of bulk breast cancer cell lines. In addition, sulforaphane inhibited mammosphere formation and the cancer cells from sulforaphane-treated mice failed to produce any tumors in recipient mice up to 33 days after implantations [23].
Metformin, a known oral hypoglycemic agent, was reported to selectively kill breast CSCs through an unknown mechanism. In this study, the combination of metformin and doxorubicin had a synergistic effect in reducing tumor mass and preventing relapse in xenograft mice [24]. In addition, Jiralerspong et al. reported a study of 2529 patients undergoing neoadjuvant chemotherapy in which the pathologic complete response rate following surgery was 24% for diabetic patients taking metformin, 8% for diabetic patients not taking metformin, and 16% for nondiabetic patients [25]. Furthermore, using both CXCR1-blocking antibodies and the small molecule inhibitor, repertaxin, respectively, demonstrated that CXCR1 selectively decreased breast CSCs in vitro and in NOD/SCID xenograft models [26].
Berberine was investigated to determine its effects on the enriched stem cell-like population of MCF-7 cells. After 72-hours, the viability of MCF-7 cells was reduced to 96%, 84%, 79%, 65%, and 56%, and the stem cell-like population was reduced to 1.4% (1.5% of MCF7 cells were the stem cell-like population cells), 1.1%, 0.9%, 0.5%, and 0.2% by 10 nM, 100 nM, 1 μM, 10 μM, and 20 μM of berberine, respectively, indicating that the stem cell-like population was being reduced more than non-stem cell-like cells based on their proportions [27].
All nine compounds identified through this project are registered with the NIH Molecular Libraries.
To date, we have identified 3 probe compounds, ML239 (Probe 1), ML245 (Probe 2), and ML243 (Probe 3). The three probes are potent inhibitors (0.5–2μM) against the Breast Cancer Stem Cell-like cells, sufficiently selective (14.7–32 fold) compared to HMLE_sh_ECad, and meet the probe criteria. ML245 is the most potent against the primary target cell line, while ML243 has the greatest selectivity towards HMLE_sh_ECad (see Table 4).
Table 4
Comparison of Breast Cancer Stem Cells: Probes 1, 2, and 3.
5. References
- 1.
- Bao S, Wu Q, McLendon RE, Hao Y, Shi Q, Hjelmeland AB, Dewhirst MW, Bigner DD, Rich JN. Glioma stem cells promote radioresistance by preferential activation of the DNA damage response. Nature. 2006;444:756–760. [PubMed: 17051156]
- 2.
- Diehn M, Cho RW, Lobo NA, Kalisky T, Dorie MJ, Kulp AN, Qian D, Lam JS, Ailles LE, Wong M, et al. Association of reactive oxygen species levels and radioresistance in cancer stem cells. Nature. 2009;458:780–783. [PMC free article: PMC2778612] [PubMed: 19194462]
- 3.
- Diehn M, Clarke MF. Cancer stem cells and radiotherapy new insights into tumor radioresistance. J Natl Cancer Inst. 2006;98:1755–1757. [PubMed: 17179471]
- 4.
- Eyler CE, Rich JN. Survival of the fittest cancer stem cells in therapeutic resistance and angiogenesis. J Clin Oncol. 2008;26:2839–2845. [PMC free article: PMC2739000] [PubMed: 18539962]
- 5.
- Li X, Lewis MT, Huang J, Gutierrez C, Osborne CK, Wu MF, Hilsenbeck SG, Pavlick A, Zhang X, Chamness GC, et al. Intrinsic resistance of tumorigenic breast cancer cells to chemotherapy. J Natl Cancer Inst. 2008;100:672–679. [PubMed: 18445819]
- 6.
- Mani SA, Guo W, Liao MJ, Eaton EN, Ayyanan A, Zhou AY, Brooks M, Reinhard F, Zhang CC, Shipitsin M, et al. The epithelial-mesenchymal transition generates cells with properties of stem cells. Cell. 2008;133:704–715. [PMC free article: PMC2728032] [PubMed: 18485877]
- 7.
- Gupta PB, Onder TT, Jiang G, Tao K, Kuperwasser C, Weinberg RA, Lander ES. Identification of selective inhibitors of cancer stem cells by high-throughput screening. Cell. 2009;138:645–659. [PMC free article: PMC4892125] [PubMed: 19682730]
- 8.
- Dontu G, Abdallah WM, Foley JM, Jackson KW, Clarke MF, Kawamura MJ, Wicha MS. In vitro propagation and transcriptional profiling of human mammary stem/progenitor cells. Genes Dev. 2003;17:1253–1270. [PMC free article: PMC196056] [PubMed: 12756227]
- 9.
- Carmody LC, Germain A, Morgan B, Burhlage S, VerPlank L, Munoz Be, Feng Y, Gupta PB, Lander ES, Palmer M, Schreiber SL. Identification of selective Breast Cancer Stem Cell Inhibitors. Molecular Library Probe Center Network Probe Report. 2011.
- 10.
- Onder TT, Gupta PB, Mani SA, Yang J, Lander ES, Weinberg RA. Loss of E-cadherin promotes metastasis via multiple downstream transcriptional pathways. Cancer Res. 2008;68:3645–3654. [PubMed: 18483246]
- 11.
- Dawson PA, Pirlo KJ, Steane SE, Kunzelmann K, Chien YJ, Markovich D. Molecular cloning and characterization of the mouse Na+ sulfate cotransporter gene (Slc13a4): Structure and expression. Genes Genet Syst. 2006;81:265–272. [PubMed: 17038798]
- 12.
- Perez DM. Structure-function of alpha1-adrenergic receptors. Biochem Pharmacol. 2007;73:1051–1062. [PMC free article: PMC2034198] [PubMed: 17052695]
- 13.
- Gautier T, Lagrost L. Plasma PLTP (phospholipid-transfer protein): an emerging role in ‘ reverse lipopolysaccharide transport’ and innate immunity. Biochem Soc Trans. 2011;39:984–988. [PubMed: 21787334]
- 14.
- Gloriam DE, Schioth HB, Fredriksson R. Nine new human Rhodopsin family G-protein coupled receptors: identification sequence characterisation and evolutionary relationship. Biochim Biophys Acta. 2005;1722:235–246. [PubMed: 15777626]
- 15.
- Fox BP, Kandpal RP. Invasiveness of breast carcinoma cells and transcript profile. Eph receptors and ephrin ligands as molecular markers of potential diagnostic and prognostic application. Biochem Biophys Res Commun. 2004;318:882–892. [PubMed: 15147954]
- 16.
- Stuchell MD, Garrus JE, Muller B, Stray KM, Ghaffarian S, McKinnon R, Krausslich HG, Morham SG, Sundquist WI. The human endosomal sorting complex required for transport (ESCRT-I) and its role in HIV-1 budding. J Biol Chem. 2004;279:36059–36071. [PubMed: 15218037]
- 17.
- Jin HO, Seo SK, Woo SH, Choe TB, Hong SI, Kim JI, Park IC. Nuclear protein 1 induced by ATF4 in response to various stressors acts as a positive regulator on the transcriptional activation of ATF4. IUBMB Life. 2009;61:1153–1158. [PubMed: 19946894]
- 18.
- Lu D, Choi MY, Yu J, Castro JE, Kipps TJ, Carson DA. Salinomycin inhibits Wnt signaling and selectively induces apoptosis in chronic lymphocytic leukemia cells. Proc Natl Acad Sci U S A. 2011;108:13253–13257. [PMC free article: PMC3156152] [PubMed: 21788521]
- 19.
- McKiernan E, McDermott EW, Evoy D, Crown J, Duffy MJ. The role of S100 genes in breast cancer progression. Tumour Biol. 2011;32:441–450. [PubMed: 21153724]
- 20.
- Zhou J, Zhang H, Gu P, Bai J, Margolick JB, Zhang Y. NF-kappaB pathway inhibitors preferentially inhibit breast cancer stem-like cells. Breast Cancer Res Treat. 2008;111:419–427. [PMC free article: PMC3320112] [PubMed: 17965935]
- 21.
- Zhou J, Zhang H, Gu P, Margolick JB, Yin D, Zhang Y. Cancer stem/progenitor cell active compound 8-quinolinol in combination with paclitaxel achieves an improved cure of breast cancer in the mouse model. Breast Cancer Res Treat. 2009;115:269–277. [PMC free article: PMC3320107] [PubMed: 18506619]
- 22.
- Kakarala M, Brenner DE, Korkaya H, Cheng C, Tazi K, Ginestier C, Liu S, Dontu G, Wicha MS. Targeting breast stem cells with the cancer preventive compounds curcumin and piperine. Breast Cancer Res Treat. 2010;122:777–785. [PMC free article: PMC3039120] [PubMed: 19898931]
- 23.
- Li Y, Zhang T, Korkaya H, Liu S, Lee HF, Newman B, Yu Y, Clouthier SG, Schwartz SJ, Wicha MS, et al. Sulforaphane, a dietary component of broccoli/broccoli sprouts inhibits breast cancer stem cells. Clin Cancer Res. 2010;16:2580–2590. [PMC free article: PMC2862133] [PubMed: 20388854]
- 24.
- Hirsch HA, Iliopoulos D, Tsichlis PN, Struhl K. Metformin selectively targets cancer stem cells and acts together with chemotherapy to block tumor growth and prolong remission. Cancer Res. 2009;69:7507–7511. [PMC free article: PMC2756324] [PubMed: 19752085]
- 25.
- Jiralerspong S, Palla SL, Giordano SH, Meric-Bernstam F, Liedtke C, Barnett CM, Hsu L, Hung MC, Hortobagyi GN, Gonzalez-Angulo AM. Metformin and pathologic complete responses to neoadjuvant chemotherapy in diabetic patients with breast cancer. J Clin Oncol. 2009;27:3297–3302. [PMC free article: PMC2736070] [PubMed: 19487376]
- 26.
- Ginestier C, Liu S, Diebel ME, Korkaya H, Luo M, Brown M, Wicinski J, Cabaud O, Charafe-Jauffret E, Birnbaum D, et al. CXCR1 blockade selectively targets human breast cancer stem cells in vitro and in xenografts. J Clin Invest. 2010;120:485–497. [PMC free article: PMC2810075] [PubMed: 20051626]
- 27.
- Kim JB, Ko E, Han W, Shin I, Park SY, Noh DY. Berberine diminishes the side population and ABCG2 transporter expression in MCF-7 breast cancer cells. Planta Med. 2008;74:1693–1700. [PubMed: 18951337]
- PMCPubMed Central citations
- PubChem BioAssay for Chemical ProbePubChem BioAssay records reporting screening data for the development of the chemical probe(s) described in this book chapter
- PubChem SubstanceRelated PubChem Substances
- PubMedLinks to PubMed
- Review Identification of a Selective Small-Molecule Inhibitor of Breast Cancer Stem Cells—Probe 2.[Probe Reports from the NIH Mol...]Review Identification of a Selective Small-Molecule Inhibitor of Breast Cancer Stem Cells—Probe 2.Carmody LC, Germain A, Morgan B, VerPlank L, Fernandez C, Feng Y, Perez JR, Dandapani S, Munoz B, Palmer M, et al. Probe Reports from the NIH Molecular Libraries Program. 2010
- Review Identification of a Selective Small-Molecule Inhibitor of Breast Cancer Stem Cells - Probe 1.[Probe Reports from the NIH Mol...]Review Identification of a Selective Small-Molecule Inhibitor of Breast Cancer Stem Cells - Probe 1.Carmody L, Germain A, Morgan B, VerPlank L, Fernandez C, Forbeck E, Ting A, Feng Y, Perez J, Dandapani S, et al. Probe Reports from the NIH Molecular Libraries Program. 2010
- Phenotypic high-throughput screening elucidates target pathway in breast cancer stem cell-like cells.[J Biomol Screen. 2012]Phenotypic high-throughput screening elucidates target pathway in breast cancer stem cell-like cells.Carmody LC, Germain AR, VerPlank L, Nag PP, Muñoz B, Perez JR, Palmer MA. J Biomol Screen. 2012 Oct; 17(9):1204-10. Epub 2012 Aug 30.
- Identification of a selective small molecule inhibitor of breast cancer stem cells.[Bioorg Med Chem Lett. 2012]Identification of a selective small molecule inhibitor of breast cancer stem cells.Germain AR, Carmody LC, Morgan B, Fernandez C, Forbeck E, Lewis TA, Nag PP, Ting A, VerPlank L, Feng Y, et al. Bioorg Med Chem Lett. 2012 May 15; 22(10):3571-4. Epub 2012 Jan 25.
- Cinnamides as selective small-molecule inhibitors of a cellular model of breast cancer stem cells.[Bioorg Med Chem Lett. 2013]Cinnamides as selective small-molecule inhibitors of a cellular model of breast cancer stem cells.Germain AR, Carmody LC, Nag PP, Morgan B, Verplank L, Fernandez C, Donckele E, Feng Y, Perez JR, Dandapani S, et al. Bioorg Med Chem Lett. 2013 Mar 15; 23(6):1834-8. Epub 2013 Jan 16.
- Identification of a Selective Small-Molecule Inhibitor of Breast Cancer Stem Cel...Identification of a Selective Small-Molecule Inhibitor of Breast Cancer Stem Cells - Probe 3 - Probe Reports from the NIH Molecular Libraries Program
Your browsing activity is empty.
Activity recording is turned off.
See more...