NCBI Bookshelf. A service of the National Library of Medicine, National Institutes of Health.
Probe Reports from the NIH Molecular Libraries Program [Internet]. Bethesda (MD): National Center for Biotechnology Information (US); 2010-.
The isozymes of alkaline phosphatase (AP) are capable of dephosphorylation and transphosphorylation of a wide spectrum of substrates in vitro. Their localization in proximity to the cell surface suggests that they help facilitate the movement of substances across the plasma membrane. In humans, four isozymes of APs have been identified - one tissue-nonspecific (TNAP) and three tissue-specific isozymes named according to the tissue of their predominant expression: intestinal (IAP), placental (PLAP) and germ cell (GCAP) alkaline phosphatases.
This project sought to identify isozyme and species selective inhibitors and activators of the human and mouse alkaline phosphatases. Concurrent high throughput screening (HTS) campaigns of the NIH Molecular Libraries Small Molecule Repository (MLSMR) library comprising ~355,000 compounds were completed against murine IAP (muIAP), human IAP (huIAP), TNAP and PLAP (TNAP & PLAP are only found in humans) were completed. This Center Probe Report describes the screens and structure activity relationship (SAR) elucidation that led to identification of a potent (540 nM IC50) inhibitor of the murine IAP (muIAP), with 65-fold selectivity against TNAP and >185-fold selectivity against PLAP as well as the human isoform of IAP (huIAP).
Assigned Assay Grant #: X01-MH077602-01
Screening Center Name & PI: Sanford-Burnham Medical Research Institute & John C. Reed, M.D., Ph.D.
Chemistry Center Name & PI: Sanford-Burnham Medical Research Institute & John C. Reed, M.D., Ph.D.
Assay Submitter & Institution: Sanford-Burnham Medical Research Institute & José Luis Millán (PI)
University of California, Los Angeles, School of Medicine & Jonathan Kaunitz (co-PI)
PubChem Summary Bioassay Identifier (AID): 2818
Probe Structure & Characteristics
This Center Probe Report describes a selective and murine species-specific intestinal alkaline phosphatase (muIAP) inhibitor probe ML260. Potency and selectivity characteristics are summarized for this probe in Table 1.
Table 1
Biological activity summary & AIDs for Probes.

1. Recommendations for Scientific Use of the Probe
To the present date, the scientific research community has no good selective inhibitors of mouse intestinal alkaline phosphatase (muIAP) that discriminate between the activity of the tissue-specific isozymes of AP, murine, or human in origin, such as the mouse embryonic AP, product of the Akp5 gene and global intestinal AP, product of the Akp6 locus, or the human Placental AP (PLAP) and germ cell AP (GCAP)), to assist in elucidating its functional and physiological roles. Such roles include detoxifying bacterial endotoxins and thereby acting in the establishment of gut mucosal tolerance towards the microbiota, functioning as a rate-determining step in the absorption of fatty acids, and in the regulation of duodenal surface pH and ATP concentration. This probe can be applied to the analysis of physiologic role of IAPs and other isozymes in the gut in mouse models and in the examination of the many other hydrolytic enzymes relating to ATP release mechanisms, which are otherwise obscured by the rapid hydrolysis of ATP at the cell surface. In fact, given that the mouse Akp3 (muIAP), Akp5, and Akp6 genes are syntenic and very close together in the chromosome, obtaining double knock-out (KO) mice (e.g. Akp3/Akp6 double KO, or Akp3/Akp5 double KOs) to investigate function is next to impossible. So, the ability to inhibit Akp3 (muIAP) through a chemical probe in an Akp5 or Akp6 KO mouse models will help us dissect the distinct functions of these isozymes.
The somewhat unexpected species selectivity of ML260 indeed provides a tool to elucidate the molecular interactions and structural determinants of selective inhibition in the purified enzymes.
It can also be applied to the study of the basic biology of plasma membrane fatty acid uptake and the biology of pulmonary inflammation induced by pathogens. It is hoped that scientists interested in the basic biology of human AP and those interested in translational applications of that research, such as gastrointestinal and pulmonary physiologists with interests in obesity, intestinal lipid uptake, and cystic fibrosis, will find this probe of interest.
2. Materials and Methods
2.1. Assays
Table 2 summarizes the details for the assays that drove this probe project and can be found in the “Assay Description” section under the listed AIDs in Table 2 as viewable in PubChem Bioassays.
Table 2
Summary of Assays and AIDs.
2.2. Probe Chemical Characterization
Chemical name of probe compound & structure including stereochemistry
The IUPAC name of the probe is N-(2,5-dimethylphenyl)-2-(2-oxo-2,3-dihydrobenzo[d]oxazole-6-sulfonamido)acetamide. The actual batch prepared, tested, and submitted to the MLSMR is archived as SID 124756790 corresponding to CID 50919367. It has no chiral centers. The probe molecule is not commercially available. A 20 mg sample of ML260 synthesized at SBCCG has been deposited in the MLSMR (Bio-Focus DPI) (see Probe Submission Table 3).
Table 3
Probe and Analog Submissions to MLSMR (BioFocus DPI) for muIAP Inhibitors.
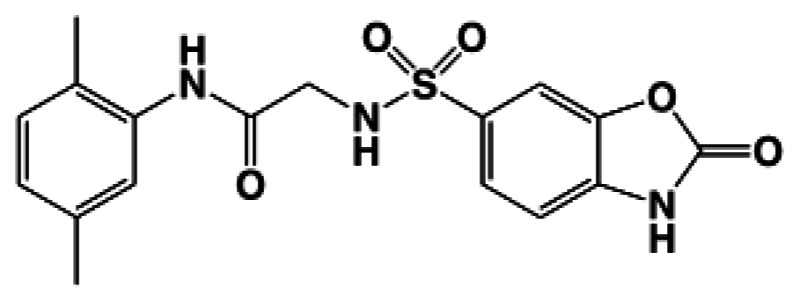
Figure 1Structure of ML260
Synthetic Scheme and Structural Verification. Probe ML260 SID 124756790 corresponding to CID 50919367 was synthesized according to Scheme 1, and Figs. 2 and 3 provide 1H NMR and LC-MS structure proof, respectively.

Scheme 1
Synthesis of ML260. conditions: a. dichloromethane, triethylamine, (88% yield); b. trifluoroacetic acid, dichloromethane, 0°C warm to RT (100% yield); c. EDC, HOBT, NMM, DMF, (55%)
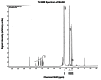
Figure 2
1H NMR Spectrum of ML260.

Figure 3
LC-MS for ML260. (Reverse phase C18 column: isocratic)
Solubility and Stability of probe in PBS at room temperature
The stability and solubility of ML260 was investigated in phosphate-buffered saline (PBS) at room temperature (Figure 4). A comparison of the %parent compound remaining at various times indicates that the compound is very stable for much more than 48 hrs.
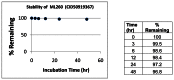
Figure 4
Stability of ML260 in 1:1 acetonitrile:PBS at room temperature.
MLS# that were submitted to the SMR collection
Samples of the probe and five analogs synthesized at SBCCG were submitted to MLSMR (Table 3).
2.3. Probe Preparation
(compound numbering according to Scheme 1)
Preparation of tert-butyl 2-(2-oxo-2,3-dihydrobenzo[d]oxazole-6-sulfonamido)acetate

2-oxo-2,3-dihydrobenzo[d]oxazole-6-sulfonyl chloride 1 (2.33 g, 0.01 mol) was dissolved in 50 mL of methylene chloride containing 1 mL of triethylamine. The solution was cooled to 0°C in an ice bath and a solution of tert-butyl 2-aminoacetate (1.31 g, 0.01 mol) in 20 mL of methylene chloride was slowly added. The reaction was warmed to room temperature and when the reaction was determined to be complete by TLC, approximately 1-hour, the reaction mixture was poured into 100 mL of water, washed successively 100 mL of saturated sodium bicarbonate, 100 mL of brine, and the resultant organic phase was then dried over anhydrous sodium sulfate, filtered, and concentrated under reduced pressure. The resulting solid was chromatographed on silica gel and eluted with methylene chloride to yield 2.90 g of compound 2 (88 % yield), MS ESI m/z 329 [M+H].
Preparation of 2-(2-oxo-2,3-dihydrobenzo[d]oxazole-6-sulfonamido)acetic acid

Tert-butyl 2-(2-oxo-2,3-dihydrobenzo[d]oxazole-6-sulfonamido)acetate 2 (2.90g, 8.8 mmol) was dissolved in 100 mL of methylene chloride and the solution was cooled to 0°C in an ice bath. At his point 10 mL of trifluoracetic acid was added and the reaction was allowed to warm to room temperature. When the reaction was determined to be complete by TLC, approximately 2-hours, the solvent was removed under reduced pressure. At this point, 50 mL of toluene and 50 mL of methylene chloride was added to the crude reaction mixture and the solvent was removed under reduced pressure. This procedure was repeated two more times and the flask was put under high vacuum for 30 minutes. The grey solid 2-(2-oxo-2,3-dihydrobenzo[d]oxazole-6-sulfonamido)acetic acid 3 (2.4 g, 100 %) was not purified further and was used as isolated in the next step. MS ESI m/z 273 [M+H].
Preparation of N-(2,5-dimethylphenyl)-2-(2-oxo-2,3-dihydrobenzo[d]oxazole-6-sulfonamido) acet-amide

2-(2-oxo-2,3-dihydrobenzo[d]oxazole-6-sulfonamido)acetic acid 3 (0.27 g, 1 mmol) was dissolved in 3 mL of dimethylformamide and EDC (0.21g, 1.1 mol), HOBT (0.17g, 1.1 mmol) and 0.5 mL of NMM was added to the solution. The reaction mixture was stirred for 30 minutes at room temperature and then a solution of 2,5-dimethylaniline 4 (0.24g 2 mmol) in 1 mL of dimethylformamide was added. The reaction was stirred overnight at room temperature and then the solvent was removed under reduced pressure. The residue was dissolved in 30 mL of ethyl acetate and the organic layer was poured into 100 mL of water, washed successively 50 mL of saturated sodium bicarbonate, 50 mL of 1 N HCl, 50 mL of brine, and the resultant organic phase was then dried over anhydrous sodium sulfate, filtered, and concentrated under reduced pressure. The resulting solid was chromatographed on silica gel and eluted with methylene chloride: ethyl acetate using a gradient of 100:1 to 90:10, to yield N-(2,5-dimethylphenyl)-2-(2-oxo-2,3-dihydrobenzo[d]oxazole-6-sulfonamido)acetamide ML260 (0.20 g, 55 % yield), 1H NMR (400 MHz, CDCl3) δ 9.18 (s, 1H), 8.08 (b, 1H), 7.71 (s, 1H), 7.67 (d, J = 6.7 Hz, 1H), 7.24 (d, J = 6.7 Hz, 1 H), 7.05 (d, J = 6.7 Hz, 1H), 6.98 (S, 1H), 6.84 (d, J = 6.7 Hz, 1H),3.66 (s, 2H), 2.49 (s, 3H), 2.19 (s, 3H), MS ESI m/z 376 [M+H].
3. Results
3.1. Dose Response Curves for Probe
The multiple dose response titrations in Figure 5 (on the right) of the probe ML260 against muIAP, TNAP and PLAP highlight the 65-fold selectivity of it against TNAP and the more than 185-fold selectivity against PLAP.
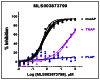
Figure 5
Multiple dose response titrations of ML260.
3.2. Cellular Activity
This probe is an in vitro inhibitor, the assay providers’ (PI and co-PI) respective laboratories will conduct further profiling and will determine the translation of these activities in further in vitro then in vivo models.
3.3. Profiling Assays
The probe was evaluated in a detailed in vitro pharmacology panel as shown in Table 4.
Table 4
Summary of in vitro ADME Properties of Novel Selection of muIAP Inhibitor Probe ML260.
ML260 is very soluble in aqueous media at pH 5.0/6.2/7.4 at more than 400-fold higher concentration than the potency of the probe for muIAP (IC50 = 540 nM) at all pH’s tested.
The PAMPA (Parallel Artificial Membrane Permeability Assay) assay is used as an in vitro model of passive, transcellular permeability. An artificial membrane immobilized on a filter is placed between a donor and acceptor compartment. At the start of the test, drug is introduced in the donor compartment. Following the permeation period, the concentration of drug in the donor and acceptor compartments is measured using UV spectroscopy. Consistent with the predicted LogP, ML260 is moderately permeable in this assay.
Plasma Protein Binding is a measure of a drug’s efficiency to bind to the proteins within blood plasma. The less bound a drug is, the more efficiently it can traverse cell membranes or diffuse. Highly plasma protein bound drugs are confined to the vascular space, thereby having a relatively low volume of distribution. In contrast, drugs that remain largely unbound in plasma are generally available for distribution to other organs and tissues. ML260 shows high binding to plasma proteins in human plasma at 1 μM and at 10 μM concentration. We were unable to determine a value for protein binding in mouse plasma, since the apparent amount of ML260 in the plasma chamber was lower than in the reference chamber, which is consistent with its lower stability in mouse plasma.
Plasma Stability is a measure of the stability of small molecules and peptides in plasma and is an important parameter, which strongly can influence the in vivo efficacy of a test compound. Drug candidates are exposed in plasma to enzymatic processes (proteinases, esterases), and they can undergo intramolecular rearrangement or bind irreversibly (covalently) to proteins. ML260 shows excellent stability in human and poor stability in mouse plasma. Mouse plasma is known to contain higher levels of these enzymes and additional enzymes, which may account for this poor stability.
The microsomal stability assay is commonly used to rank compounds according to their metabolic stability. This assay addresses the pharmacologic question of how long the parent compound will remain circulating in plasma within the body. ML260 shows relatively poor stability in human liver homogenates, and good stability in mouse liver homogenates that may improve the utility of this probe in in vivo rodent models with respect to hepatic metabolism, though this may be confounded with its apparently poor plasma stability in mice.
4. Discussion
The isozymes of alkaline phosphatase (AP; orthophosphoric monoester phosphohydrolase, EC 3.1.3.1) are ectoenzymes anchored onto the cytoplasmic membrane via a phosphatidylinositol glycan moiety1,2. They are capable of catalyzing dephosphorylation and transphosphorylation reactions of a wide spectrum of substrates in vitro2. These selective probe(s) will be useful in elucidating the functional roles of human and mouse IAPs in 1) detoxifying bacterial endotoxins and thereby acting in the establishment of gut mucosal tolerance towards the microbiota3; 2) their function as a rate-limiting step in the absorption of fatty acids4–6; and 3) in its role in influencing bicarbonate secretion in the gut7. In addition, high potency selective inhibitors of IAP isozymes would facilitate studies of ecto-purinergic regulation of duodenal bicarbonate secretion, examining the many ATP phosphohydrolases present in the intestinal brush border, which would enable the study of ATP release mechanisms8. With respect to these latter three biological questions the role of IAP in gut biology, the most relevant selectivities towards potential “off-target” activities are for the highly expressed (and readily available) isozymes, TNAP and PLAP, As most initial studies would be in mice, we chose to drive probe development through the potency against muIAP and selectivity against huIAP, TNAP and PLAP as shown in Table 1.
4.1. Comparison to Existing Art and How the New Probe is an Improvement
During the Molecular Library Screening Center Network (MLSCN) pilot phase or the Molecular Libraries Initiative (MLI), we developed a triazole probe ML038 (CID 294673, Figure 6) (https://mli.nih.gov/mli/?dl_id=712) that was more selective for the human IAP instead of TNAP (795 nM IC50; 6.5-fold) or PLAP (4,810 nM IC50; 39-fold) and exhibited a novel mode of action: uncompetitive versus the phosphate-donor substrate. While this probe is the most potent human IAP inhibitor (122 nM in the huIAP luminescent assay) known thus far, it represents only one scaffold and the species specificity was not examined.
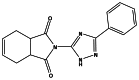
Figure 6
ML038 (CID 2946732).
From a recent survey of the literature and SciFinder patent search, there are very few examples of intestinal alkaline phosphatase (IAP) inhibitors (and no activators) that show selectivity for the target over the other alkaline phosphatase isozymes (PLAP, TNAP) in the literature. A recent disclosure of modulators, i.e., activators and inhibitors, of Intestinal Alkaline Phosphatase (IAP) describes classes of molecules that can be used for treating bacterial infections of the intestinal tract and methods for maintaining the health of the intestinal tract using IAP activators. However, no selectivity data is presented for these compounds. Further disclosed are methods to assist in weight gain of emaciated patients and those having reduced or negligible fat absorption using IAP inhibitors (US2010/0016313 A1). A representative molecule from this patent is illustrated in Figure 7.

Figure 7
Representative molecule from patent US2010/0016313 A1.
The probe described in this report represents a novel, chemically distinct, first-in-class inhibitor probe ML260 of the mouse IAP isozyme, that is the most potent, sub-micromolar (muIAP IC50 = 540 nM), and selective inhibitor reported for the enzyme from this species. As a comparison, its potency (muIAP IC50 = 540 nM) for the mouse IAP, is comparable to that of the previous MLSCN probe ML038 (huIAP IC50 = 122 nM) for the human enzyme, however it represents significant improvements in selectivity against the other isozymes, TNAP (65-fold ML260 vs. 6.5-fold ML038) and PLAP (>185-fold ML260 vs. 39-fold ML038). However, it should be noted that this probe is highly selective for the mouse vs. the human isoform. Our lab (Dr. Millán) has shown that the phylogenetic analysis shows that the mouse isozymes are closer in homology to each other than to the human isozymes [5], so our finding of such a species selective probe for muIAP validates this on an enzyme functional level. Also, the dihydrobenzo[d]oxazole-6-sulfonamido-acetamide core of ML260 represents a completely different chemical scaffold as compared to the triazole scaffold of ML038, so it will potentially offer a pharmacophore with different ADME/T PK properties than ML038. Indeed ML260 has excellent solubility and stability properties.
5. References
- 1.
- Low MG, Prasad AR. A phospholipase D specific for the phosphatidylinositol anchor of cell-surface proteins is abundant in plasma. Proc Natl Acad Sci USA. 1988;85:980–984. [PMC free article: PMC279684] [PubMed: 3422494]
- 2.
- Millan JL. Alkaline Phosphatases: Structure, substrate specificity and functional relatedness to other members of a large super family of enzymes. In Purinergic Signalling. 2006;2(2):335–341. [Review] [PMC free article: PMC2254479] [PubMed: 18404473]
- 3.
- Goldberg RF, Austen WG Jr, Zhang X, Munene G, Mostafa G, Biswas S, McCormack M, Eberlin K, Nguyen JT, Tatlidede HS, Warren HS, Malo MS, Narisawa S, Millán JL, Hodin RA. Intestinal alkaline phosphatase is a gut defense factor maintained by enteral nutrition. Proc Natl Acad Sci USA. 2008;105:3551–3556. [PMC free article: PMC2265168] [PubMed: 18292227]
- 4.
- Narisawa S, Huang L, Iwasaki A, Hasegawa H, Alpers DH, Millán JL. Accelerated fat absorption in intestinal alkaline phosphatase knockout mice. Mol Cell Biol. 2003;23:7525–7530. [PMC free article: PMC207564] [PubMed: 14560000]
- 5.
- Narisawa S, Hoylaerts MF, Doctor KS, Fukuda MN, Alpers DH, Millán JL. A novel phosphatase upregulated in Akp3 knockout mice. Am J Physiol Gastrointest Liver Physiol. 2007;293:1068–77. [PubMed: 17901166]
- 6.
- Nakano T, Inoue I, Koyama I, Kanazawa K, Nakamura K, Narisawa S, Tanaka K, Akita M, Masuyama T, Seo M, Hokari S, Katayama S, Alpers DH, Millán JL, Komoda T. Disruption of the murine intestinal alkaline phosphatase gene (Akp3) impairs lipid transcytosis and induces visceral fat accumulation and hepatic steatosis. Am J Physiol Gastrointest Liver Physiol. 2007;292:1439–1449. [PubMed: 17332477]
- 7.
- Akiba Y, Watanabe C, Mizumori M, Kaunitz JD. Luminal L-glutamate enhances duodenal mucosal defense mechanisms via multiple glutamate receptors in rats. Am J Physiol Gastrointest Liver Physiol. 2009;297(4):G781–791. [PMC free article: PMC2763813] [PubMed: 19643955]
- 8.
- Mizumori M, Ham M, Guth PH, Engel E, Kaunitz JD, Akiba Y. Intestinal alkaline phosphatase regulates protective surface microclimate pH in rat duodenum. J Physiol. 2009;587(Pt 14):3651–63. Epub 2009 May 18. [PMC free article: PMC2742288] [PubMed: 19451200]
- PMCPubMed Central citations
- PubChem BioAssay for Chemical ProbePubChem BioAssay records reporting screening data for the development of the chemical probe(s) described in this book chapter
- PubChem SubstanceRelated PubChem Substances
- PubMedLinks to PubMed
- Modulators of intestinal alkaline phosphatase.[Methods Mol Biol. 2013]Modulators of intestinal alkaline phosphatase.Bobkova EV, Kiffer-Moreira T, Sergienko EA. Methods Mol Biol. 2013; 1053:135-44.
- Identification of a selective inhibitor of murine intestinal alkaline phosphatase (ML260) by concurrent ultra-high throughput screening against human and mouse isozymes.[Bioorg Med Chem Lett. 2014]Identification of a selective inhibitor of murine intestinal alkaline phosphatase (ML260) by concurrent ultra-high throughput screening against human and mouse isozymes.Ardecky RJ, Bobkova EV, Kiffer-Moreira T, Brown B, Ganji S, Zou J, Pass I, Narisawa S, Iano FG, Rosenstein C, et al. Bioorg Med Chem Lett. 2014 Feb 1; 24(3):1000-1004. Epub 2013 Dec 19.
- Review Placental Alkaline Phosphatase (PLAP) Luminescent HTS assay - Probe 2.[Probe Reports from the NIH Mol...]Review Placental Alkaline Phosphatase (PLAP) Luminescent HTS assay - Probe 2.Lanier M, Cashman J, Sergienko E, Garcia X, Brown B, Rascon J, Narisawa S, Simao AM, Millán JL, Stonich D, et al. Probe Reports from the NIH Molecular Libraries Program. 2010
- Review Placental Alkaline Phosphatase (PLAP) Luminescent HTS assay - Probe 1.[Probe Reports from the NIH Mol...]Review Placental Alkaline Phosphatase (PLAP) Luminescent HTS assay - Probe 1.Sergienko E, Garcia X, Brown B, Rascon J, Narisawa S, Simao AM, Millán JL, Stonich D, Su Y, Khan P, et al. Probe Reports from the NIH Molecular Libraries Program. 2010
- Structural evidence of functional divergence in human alkaline phosphatases.[J Biol Chem. 2002]Structural evidence of functional divergence in human alkaline phosphatases.Le Du MH, Millan JL. J Biol Chem. 2002 Dec 20; 277(51):49808-14. Epub 2002 Oct 7.
- A Selective Murine Intestinal Alkaline Phosphatase (muIAP) Inhibitor - Probe Rep...A Selective Murine Intestinal Alkaline Phosphatase (muIAP) Inhibitor - Probe Reports from the NIH Molecular Libraries Program
Your browsing activity is empty.
Activity recording is turned off.
See more...