An introduction to epigenetic signature analysis and comparison of gene-specific genome-wide methylation analysis and epigenetic modifications to specific regions of the genome
I. Introduction to Epigenetic Modifications
All human nucleated cells have a nuclear genome (sequence of DNA nucleotides) that encodes the proteins and other gene products responsible for the development of a single-cell zygote into an embryo and then a complex multicellular organism. This developmental process requires that the nuclear genome control the following:
- The timing of nuclear gene expression during embryonic development
- The location of nuclear gene expression to enable the differentiation of tissues and organs with specialized functions
Control over timing and location of gene expression occurs through epigenetic modifications – chemical alterations to DNA nucleotides or proteins that control gene expression but do not alter the DNA sequence itself. Histones, the proteins around which DNA is wound, are often targets for modifications that allow accessibility of the DNA for transcription. (See Table 1 for mechanisms of known epigenetic modifications in normal human cells.)
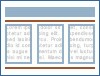
Table 1.
Mechanisms of Known Epigenetic Modifications in Normal Human Development
II. Epigenetic Modifications to Specific Regions of the Genome
Epigenetic modifications that occur early in embryogenesis to specific regions of the genome result in imprinting, the process by which maternally and paternally derived regions of chromosomes are uniquely chemically modified, leading to different expression of a certain gene or genes depending on their parental origin. Patterns of gene expression and repression vary between imprinted regions on a chromosome. DNA methylation is thought to be the principal mechanism of imprinting.
Genetic Alterations That Affect Epigenetic Modifications to Specific Regions of the Genome
Disease-associated alterations affecting methylation patterns in specific regions of the genome (see Table 2) include:
- Disruption of normal imprinting at a specific chromosome locus (e.g., heterozygous deletions or duplications of an imprinted region, uniparental disomy, and pathogenic variants that alter an imprinting control region, thereby disrupting imprint reprogramming during gametogenesis);
- Hypermethylation (silencing) of an abnormally expanded repeat region;
- Acquired promoter methylation of tumor suppressor genes; and
- Skewed X-chromosome inactivation in females due to or in the presence of a disease-causing variant on one X chromosome.
An assay designed to detect the DNA methylation pattern at a specific chromosome locus (e.g., methylation-sensitive multiplex ligation probe analysis [MS-MLPA], methylation-sensitive quantitative PCR [MS-qPCR], Southern blotting using methyl-sensitive DNA restriction enzymes) is necessary to identify epigenetic imprinting alterations. Identification of an epigenetic imprinting alteration typically requires the clinician to suspect a specific imprinting disorder and order the appropriate diagnostic test:
- First-tier testing (ordered based on clinical suspicion) is often a locus-specific DNA methylation assay to determine if an individual has a disorder caused by an abnormal methylation pattern at a specific chromosome locus (e.g., Prader-Willi/Angelman DNA methylation panel).
- Second-tier testing may be necessary to identify the cause of the abnormal methylation pattern at a specific chromosome locus (e.g., FISH testing to identify deletion of the Prader-Willi critical region).
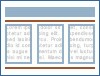
Table 2.
Disorders with an Abnormal DNA Methylation Pattern at a Specific Chromosome Locus
III. Gene-Specific Genome-Wide DNA Methylation Analysis (Epigenetic Signature Analysis)
In contrast to locus-specific DNA methylation analysis, an assay has been developed that detects the DNA methylation pattern at ~1000 different loci across the genome of a specific cell type (e.g., leukocytes). The genome-wide DNA methylation pattern in control individuals was compared to the genome-wide DNA methylation pattern in individuals with disorders known to be associated with alteration of genes that regulate DNA methylation. A unique gene-specific genome-wide DNA methylation pattern ("epigenetic signature") was identified for each of several single-gene disorders that could be distinguished from other known single-gene epigenetic signatures and the reference genome-wide DNA methylation pattern (see Table 3) [Kernohan et al 2016, Butcher et al 2017, Aref-Eshghi et al 2019].
Of note, determination of the so-called "epigenetic signature" for a specific single-gene disorder required DNA methylation analysis of a large number of individuals with known pathogenic variants in a specific gene responsible for the genome-wide epigenetic modifications. This led to the development of epigenetic signature analysis as a method that compares the genome-wide DNA methylation pattern from an individual with the altered DNA methylation pattern characteristic of each gene listed in Table 3 in a specific cell type (e.g., leukocytes).
To date, epigenetic signature analysis may be useful as a second-tier test when first-tier gene-specific molecular genetic testing has not established a molecular diagnosis. Epigenetic signature analysis is not appropriate as a first-tier test due to the limited number of single-gene disorders with an identified epigenetic signature, to date. In addition, although identification of an epigenetic signature characteristic of one of the genes listed in Table 3 is suggestive of a diagnosis, further gene-specific molecular genetic testing to identify a causative pathogenic variant is recommended to confirm the diagnosis and allow the appropriate family studies.
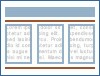
Table 3.
Genes in Which Pathogenic Variants Result in a Gene-Specific Genome-Wide Epigenetic Signature Identified on DNA Methylation Analysis of a Blood Sample
Examples of Clinical Use of Gene-Specific Genome-Wide Epigenetic Signature Analysis
An individual with clinical features suggestive of CHD7-related disorder (e.g., CHARGE syndrome) without an identified CHD7 pathogenic variant was found to have a gene-specific genome-wide DNA methylation pattern consistent with the epigenetic signature of CHD7 disorder – further supporting (but not confirming) the clinical diagnosis.
Another individual with a CHD7 variant of uncertain significance (VUS) was found to have a gene-specific genome-wide DNA methylation pattern consistent with the epigenetic signature of ADNP-related intellectual disability and autism spectrum disorder, a diagnosis subsequently confirmed by molecular genetic testing [Aref-Eshghi et al 2019].
What Are the Clinical Uses of Gene-Specific Genome-Wide Epigenetic Signature Analysis in Single-Gene Disorders?
Gene-specific genome-wide epigenetic signature analysis can suggest a molecular diagnosis in individuals with clinical features of one of the disorders in Table 3 when:
- A VUS has been identified on molecular genetic testing;
- A pathogenic variant was not identified because the molecular genetic testing methodology was not designed to detect variants such as large duplications and/or deletions, noncoding variants, or mosaic variants;
- A pathogenic variant was not identified in an individual with clinical features suggestive of more than one disorder listed in Table 3 (e.g., CHD7 disorder and Kabuki syndrome) [Butcher et al 2017].
What Are the Current Limitations of Gene-Specific Genome-Wide Epigenetic Signature Analysis in Single-Gene Disorders?
Epigenetic signature analysis has only been reported for the diagnosis of disorders that have a known distinct genome-wide epigenetic signature (see Table 3). Additional single-gene disorders with genome-wide epigenetic signatures may exist; however, data to date have not been sufficient to delineate them.
Of note, the epigenetic signature identified in males with an X-linked disorder may or may not be present in affected or unaffected females with a heterozygous pathogenic variant associated with the disorder [Kerkhof et al 2022].
Importantly, epigenetic signature analysis requires a specific sample type as DNA methylation patterns vary by cell and tissue type. For example, DNA from amniocytes, chorionic villi, and/or fibroblasts may not be appropriate to test for a gene-specific genome-wide epigenetic signature previously characterized only in peripheral blood samples. (Because DNA banking methods do not interfere with analysis of epigenetic signature from peripheral blood, DNA banked from a blood sample can be used for gene-specific genome-wide epigenetic signature analysis previously characterized in blood samples [Hjorthaug et al 2018].)
Individuals mosaic for a disorder with a known epigenetic signature may not be identified on epigenetic signature analysis if the pathogenic variant is either absent or present at a very low level in peripheral leukocytes. Testing affected tissue may not be helpful if a known epigenetic signature has not been reported or described for the affected tissue.
Possible Future Developments
Despite identification of known mechanisms of genome-wide epigenetic modification (Table 1), DNA methylation is, to date, the only method for which sufficient data exist to enable construction of a reference genome-wide pattern of epigenetic modification. It is anticipated that in the future, unique genome-wide epigenetic signatures associated with variants in additional genes will likely be identified. New assays may also be developed to identify other mechanisms associated with gene-specific genome-wide epigenetic modification.
Revision History
- 5 December 2019 (sw) Initial posting
References
- Aref-Eshghi E, Bend EG, Colaiacovo S, Caudle M, Chakrabarti R, Napier M, Brick L, Brady L, Carere DA, Levy MA, Kerkhof J, Stuart A, Saleh M, Beaudet AL, Li C, Kozenko M, Karp N, Prasad C, Siu VM, Tarnopolsky MA, Ainsworth PJ, Lin H, Rodenhiser DI, Krantz ID, Deardorff MA, Schwartz CE, Sadikovic B. Diagnostic utility of genome-wide DNA methylation testing in genetically unsolved individuals with suspected hereditary conditions. Am J Hum Genet. 2019;104:685-700. [PMC free article: PMC6451739] [PubMed: 30929737]
- Aref-Eshghi E, Bend EG, Hood RL, Schenkel LC, Carere DA, Chakrabarti R, Nagamani SCS, Cheung SW, Campeau PM, Prasad C, Siu VM, Brady L, Tarnopolsky MA, Callen DJ, Innes AM, White SM, Meschino WS, Shuen AY, Paré G, Bulman DE, Ainsworth PJ, Lin H, Rodenhiser DI, Hennekam RC, Boycott KM, Schwartz CE, Sadikovic B. BAFopathies' DNA methylation epi-signatures demonstrate diagnostic utility and functional continuum of Coffin-Siris and Nicolaides-Baraitser syndromes. Nat Commun. 2018;9:4885. [PMC free article: PMC6244416] [PubMed: 30459321]
- Aref-Eshghi E, Kerkhof J, Pedro VP; Groupe DI France, Barat-Houari M, Ruiz-Pallares N, Andrau JC, Lacombe D, Van-Gils J, Fergelot P, Dubourg C, Cormier-Daire V, Rondeau S, Lecoquierre F, Saugier-Veber P, Nicolas G, Lesca G, Chatron N, Sanlaville D, Vitobello A, Faivre L, Thauvin-Robinet C, Laumonnier F, Raynaud M, Alders M, Mannens M, Henneman P, Hennekam RC, Velasco G, Francastel C, Ulveling D, Ciolfi A, Pizzi S, Tartaglia M, Heide S, Héron D, Mignot C, Keren B, Whalen S, Afenjar A, Bienvenu T, Campeau PM, Rousseau J, Levy MA, Brick L, Kozenko M, Balci TB, Siu VM, Stuart A, Kadour M, Masters J, Takano K, Kleefstra T, de Leeuw N, Field M, Shaw M, Gecz J, Ainsworth PJ, Lin H, Rodenhiser DI, Friez MJ, Tedder M, Lee JA, DuPont BR, Stevenson RE, Skinner SA, Schwartz CE, Genevieve D, Sadikovic B. Evaluation of DNA Methylation Episignatures for Diagnosis and Phenotype Correlations in 42 Mendelian Neurodevelopmental Disorders. Am J Hum Genet. 2020;106:356-70. [PMC free article: PMC7058829] [PubMed: 32109418]
- Burkardt DD, Zachariou A, Loveday C, Allen CL, Amor DJ, Ardissone A, Banka S, Bourgois A, Coubes C, Cytrynbaum C, Faivre L, Marion G, Horton R, Kotzot D, Lay-Son G, Lees M, Low K, Luk HM, Mark P, McConkie-Rosell A, McDonald M, Pappas J, Phillipe C, Shears D, Skotko B, Stewart F, Stewart H, Temple IK, Mau-Them FT, Verdugo RA, Weksberg R, Zarate YA, Graham JM, Tatton-Brown K. HIST1H1E heterozygous protein-truncating variants cause a recognizable syndrome with intellectual disability and distinctive facial gestalt: A study to clarify the HIST1H1E syndrome phenotype in 30 individuals. Am J Med Genet A. 2019;179:2049-55. [PubMed: 31400068]
- Butcher DT, Cytrynbaum C, Turinsky AL, Siu MT, Inbar-Feigenberg M, Mendoza-Londono R, Chitayat D, Walker S, Machado J, Caluseriu O, Dupuis L, Grafodatskaya D, Reardon W, Gilbert-Dussardier B, Verloes A, Bilan F, Milunsky JM, Basran R, Papsin B, Stockley TL, Scherer SW, Choufani S, Brudno M, Weksberg R. CHARGE and Kabuki syndromes: gene-specific DNA methylation signatures identify epigenetic mechanisms linking these clinically overlapping conditions. Am J Hum Genet. 2017;100:773-88. [PMC free article: PMC5420353] [PubMed: 28475860]
- Cherik F, Reilly J, Kerkhof J, Levy M, McConkey H, Barat-Houari M, Butler KM, Coubes C, Lee JA, Le Guyader G, Louie RJ, Patterson WG, Tedder ML, Bak M, Hammer TB, Craigen W, Démurger F, Dubourg C, Fradin M, Franciskovich R, Frengen E, Friedman J, Palares NR, Iascone M, Misceo D, Monin P, Odent S, Philippe C, Rouxel F, Saletti V, Strømme P, Thulin PC, Sadikovic B, Genevieve D. DNA methylation episignature in Gabriele-de Vries syndrome. Genet Med. 2022;24:905-14. [PubMed: 35027293]
- Hjorthaug HS, Gervin K, Mowinckel P, Munthe-Kaas MC. Exploring the influence from whole blood DNA extraction methods on Infinium 450K DNA methylation. PLoS One. 2018;13:e0208699. [PMC free article: PMC6291135] [PubMed: 30540848]
- Kerkhof J, Squeo GM, McConkey H, Levy MA, Piemontese MR, Castori M, Accadia M, Biamino E, Della Monica M, Di Giacomo MC, Gervasini C, Maitz S, Melis D, Milani D, Piccione M, Prontera P, Selicorni A, Sadikovic B, Merla G. DNA methylation episignature testing improves molecular diagnosis of Mendelian chromatinopathies. Genet Med. 2022;24:51-60. [PubMed: 34906459]
- Kernohan KD, Cigana Schenkel L, Huang L, Smith A, Pare G, Ainsworth P; Care4Rare Canada Consortium, Boycott KM, Warman-Chardon J, Sadikovic B. Identification of a methylation profile for DNMT1-associated autosomal dominant cerebellar ataxia, deafness, and narcolepsy. Clin Epigenetics. 2016;8:91. [PMC free article: PMC5011850] [PubMed: 27602171]
- Levy MA, McConkey H, Kerkhof J, Barat-Houari M, Bargiacchi S, Biamino E, Bralo MP, Cappuccio G, Ciolfi A, Clarke A, DuPont BR, Elting MW, Faivre L, Fee T, Fletcher RS, Cherik F, Foroutan A, Friez MJ, Gervasini C, Haghshenas S, Hilton BA, Jenkins Z, Kaur S, Lewis S, Louie RJ, Maitz S, Milani D, Morgan AT, Oegema R, Østergaard E, Pallares NR, Piccione M, Pizzi S, Plomp AS, Poulton C, Reilly J, Relator R, Rius R, Robertson S, Rooney K, Rousseau J, Santen GWE, Santos-Simarro F, Schijns J, Squeo GM, St John M, Thauvin-Robinet C, Traficante G, van der Sluijs PJ, Vergano SA, Vos N, Walden KK, Azmanov D, Balci T, Banka S, Gecz J, Henneman P, Lee JA, Mannens MMAM, Roscioli T, Siu V, Amor DJ, Baynam G, Bend EG, Boycott K, Brunetti-Pierri N, Campeau PM, Christodoulou J, Dyment D, Esber N, Fahrner JA, Fleming MD, Genevieve D, Kerrnohan KD, McNeill A, Menke LA, Merla G, Prontera P, Rockman-Greenberg C, Schwartz C, Skinner SA, Stevenson RE, Vitobello A, Tartaglia M, Alders M, Tedder ML, Sadikovic B. Novel diagnostic DNA methylation episignatures expand and refine the epigenetic landscapes of Mendelian disorders. HGG Adv. 2021;3:100075. [PMC free article: PMC8756545] [PubMed: 35047860]
- Mulchandani S, Bhoj EJ, Luo M, Powell-Hamilton N, Jenny K, Gripp KW, Elbracht M, Eggermann T, Turner CL, Temple IK, Mackay DJ, Dubbs H, Stevenson DA, Slattery L, Zackai EH, Spinner NB, Krantz ID, Conlin LK. Maternal uniparental disomy of chromosome 20: a novel imprinting disorder of growth failure. Genet Med. 2016;18:309-15. [PubMed: 26248010]
- Smith AM, LaValle TA, Shinawi M, Ramakrishnan SM, Abel HJ, Hill CA, Kirkland NM, Rettig MP, Helton NM, Heath SE, Ferraro F, Chen DY, Adak S, Semenkovich CF, Christian DL, Martin JR, Gabel HW, Miller CA, Ley TJ. Functional and epigenetic phenotypes of humans and mice with DNMT3A Overgrowth Syndrome. Nat Commun. 2021;12:4549. [PMC free article: PMC8316576] [PubMed: 34315901]
- Yokotsuka-Ishida S, Nakamura M, Tomiyasu Y, Nagai M, Kato Y, Tomiyasu A, Umehara H, Hayashi T, Sasaki N, Ueno SI, Sano A. Positional cloning and comprehensive mutation analysis identified a novel KDM2B mutation in a Japanese family with minor malformations, intellectual disability, and schizophrenia. J Hum Genet. 2021;66:597-606. [PubMed: 33402700]
Publication Details
Author Information and Affiliations
Seattle, Washington
Emory University School of Medicine
Atlanta, Georgia
Publication History
Initial Posting: December 5, 2019; Last Revision: June 30, 2022.
Copyright
GeneReviews® chapters are owned by the University of Washington. Permission is hereby granted to reproduce, distribute, and translate copies of content materials for noncommercial research purposes only, provided that (i) credit for source (http://www.genereviews.org/) and copyright (© 1993-2024 University of Washington) are included with each copy; (ii) a link to the original material is provided whenever the material is published elsewhere on the Web; and (iii) reproducers, distributors, and/or translators comply with the GeneReviews® Copyright Notice and Usage Disclaimer. No further modifications are allowed. For clarity, excerpts of GeneReviews chapters for use in lab reports and clinic notes are a permitted use.
For more information, see the GeneReviews® Copyright Notice and Usage Disclaimer.
For questions regarding permissions or whether a specified use is allowed, contact: ude.wu@tssamda.
Publisher
University of Washington, Seattle, Seattle (WA)
NLM Citation
Wallace SE, Mirzaa GM, Bean LJH. Resources for Genetics Professionals — Epigenetic Signature Analysis. 2019 Dec 5 [Updated 2022 Jun 30]. In: Adam MP, Feldman J, Mirzaa GM, et al., editors. GeneReviews® [Internet]. Seattle (WA): University of Washington, Seattle; 1993-2024.